The homunculus is one of the most iconic images in neurology and neuroscience. Usually visualised as a series of disproportionately sized body parts splayed across a section of the brain, it shows how the body is systematically mapped onto the sensory and motor cortices, representing the proportion of brain tissue devoted to each part of the body.
This image has not only had a long-lasting impact on neurosurgical practice and basic brain research, but has also entered the public imagination, with three-dimensional clay models consisting of an enormous head and outsized hands attached to a tiny torso, on display at the Natural History Museum in London, and elsewhere.
The groundbreaking work that led to the homunculus was a major advance in our understanding of the structure and function of the brain, and the homunculus itself revolutionised the art of medical illustration. Yet modern research suggests that the homunculus is far more complex than originally thought, and some argue that it is incorrect and needs to be radically revised.
The homunculus – meaning little man – is the brainchild of the Canadian neurosurgeon Wilder Penfield (1891-1976), who co-founded the Montreal Neurological Institute at McGill University in 1934 and became its first director. There, he developed a pioneering technique for identifying, and then surgically removing, abnormal brain tissue causing epileptic seizures. Using this method over the course of his career, he and his colleagues produced early detailed maps of the functions of various regions of the cerebral cortex.
Most epileptic patients respond well to anti-convulsant drugs, but for those who do not, and whose seizures become frequent, severe and debilitating, brain surgery is a last-resort treatment. Penfield’s technique involved using an electrode to electrically stimulate the surface of the patient’s brain; crucially, they remained fully conscious on the operating table during the procedure, so that the patient could describe the effects of the stimulation. This enabled Penfield to cut out, or resect, the tissue causing the seizures without damaging neighbouring tissue involved in functions such as movement and language.
With the patient’s scalp anaesthetised and their skull opened, Penfield applied small electrical currents to the exposed surface of his patient’s brain. Because the patient remained fully conscious, Penfield could not only observe the movements evoked by stimulation of a specific area, but also ask them about the sensations and perceptions they experienced.
Stimulation of the top of the brain evoked movement or sensation in the hip and torso
Penfield operated on more than 1,000 patients throughout the 1930s and ’40s, and thus comprehensively ‘mapped’ the function of each area of the cerebral cortex. Electrical stimulation of some regions elicited the recall of long-lost memories; others triggered musical or olfactory hallucinations, famously causing one patient to report: ‘I smell burnt toast!’
His most important discovery, however, was the organisation of the sensory and motor cortices, two narrow, adjacent strips of tissue that run down from the top to the bottom of the brain on either side of the central sulcus, a deep fissure separating the frontal and parietal lobes.
Here, stimulation in front of the fissure evoked small movements or muscle twitches in specific parts of the body, and stimulation just behind it evoked sensations instead. Importantly, the body appeared to be mapped in a highly organised manner in both of these regions, such that stimulation of adjacent patches in either evoked movements or sensations in adjacent body parts on the opposite side of the body.
Thus, stimulation of the top of the brain evoked movement or sensation in the hip and torso, and stimulation progressively further down along the outer surface elicited responses first in the shoulder, arm, elbow, forearm, and then the wrist. Finally, there was a large patch of both strips of tissue devoted to the hand, with each finger represented individually, and another large patch devoted to the face, tongue and throat. Crucially, although the precise size and location of the tissue devoted to each body part differed between patients, the sequence of responses elicited by progressive stimulations from the top to the bottom of the brain was always the same.
During each procedure, Penfield would place small numbered stickers on the patient’s brain, and take note of the response evoked by electrical stimulation of that particular patch of tissue (see figure below):
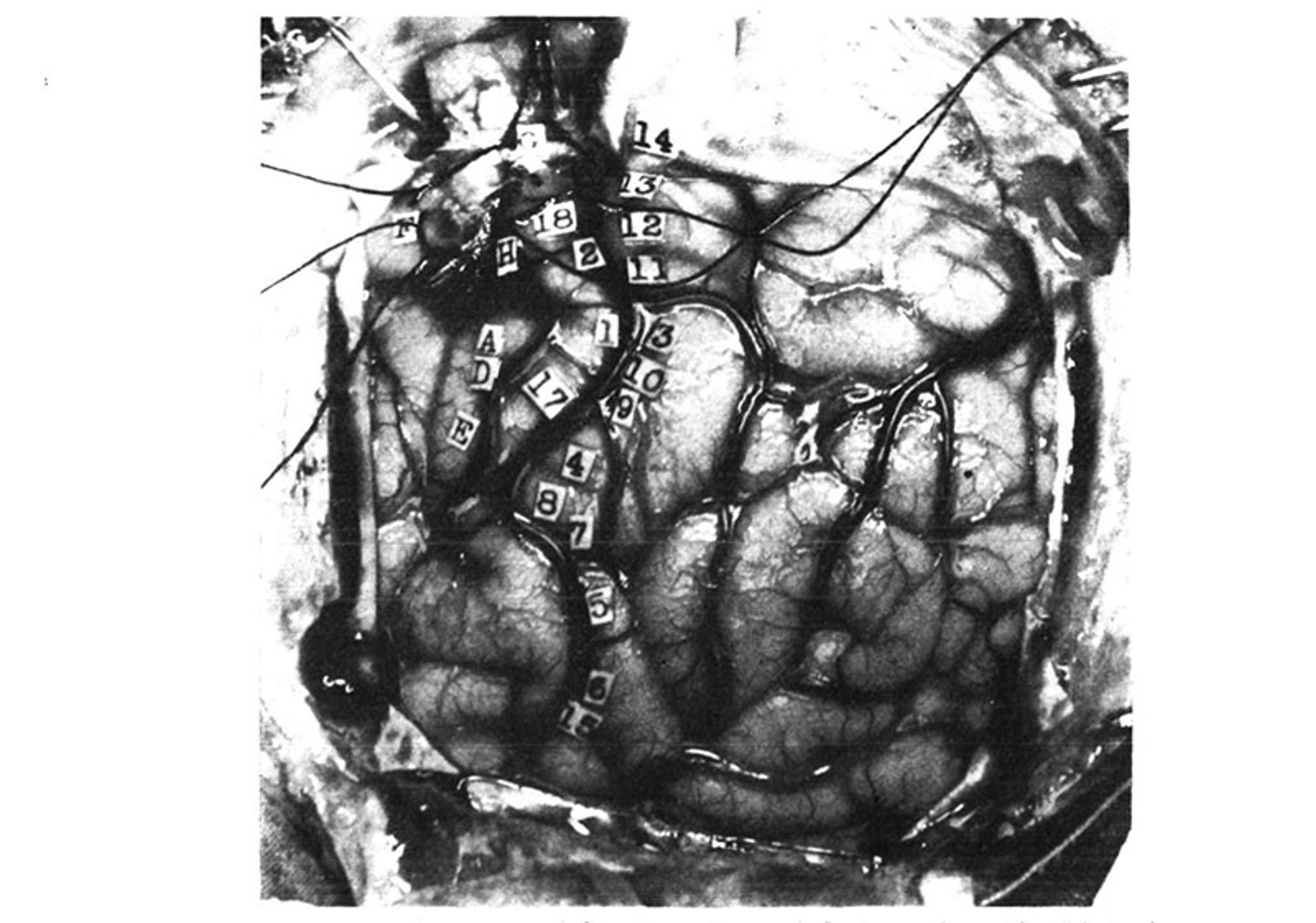
From Wilder Penfield and Edwin Boldrey’s 1937 paper. American Neurological Association
14. Tingling from the knee down to the right foot, no numbness.
13. Numbness all down the right leg, did not include the foot.
12. Numbness over the wrist, lower border, right side.
11. Numbness in the right shoulder.
3. Numb feeling in hand and forearm up to just above the forearm.
10. Tingling feeling in the fifth or little finger.
9. Tingling in first three fingers.
4. Felt like a shock and numbness in all four fingers but not in the thumb.
8. Felt sensation of movement in the thumb; no evidence of movement could be seen.
7. Same as 8.
5. Numbness in the right side of the tongue.
6. Tingling feeling in the right side of the tongue, more at the tip.
15. Tingling in the tongue, associated with up and down vibratory movements.
16. Numbness, back of tongue, mid-line.
Precentral gyrus from above down: –
(G) Flexion of knee.
18. Slight twitching of arm and hand like a shock, and felt as if he wanted to move them.
2. Shrugged shoulders upwards; did not feel like an attack.
(H) Clonic movement of right arm, shoulders, forearm, no movement in trunk.
(A) Extreme flexion of wrist, elbow and hand.
(D) Closure of hand and flexion of his wrist, like an attack.
17. Felt as if he were going to have an attack, flexion of arms and forearms, extension of wrist.
(E) Slight closure of hand; stimulation followed by local flushing of brain; this was repeated with the strength at 24. Flushing was followed by pallor for a few seconds.
(B) Patient states that he could not help closing his right eye but he actually closed both.
(C) Made a little noise; vocalisation. This was repeated twice. Patient says he could not help it. It was associated with movement of the upper and lower lips, equal on the two sides …
It is these findings that are immortalised in visual form as the homunculus, which first appeared in Penfield’s paper ‘Somatic Motor and Sensory Representation in the Cerebral Cortex of Man as Studied by Electrical Stimulation’ (1937), co-authored with Edwin Boldrey. The findings reveal that the motor and sensory cortices are organised in such a way that there is a point-for-point correspondence of body parts to specific regions of brain tissue, with adjacent body parts ‘represented’ by adjacent patches of tissue.
This organisation is referred to as ‘somatotopy’, and it is widely considered to be a fundamental principle of brain structure and function. Furthermore, Penfield’s technique, which came to be known as ‘the Montreal Procedure’, is still used today. Several years ago, for example, the violinist Dagmar Turner played her instrument throughout a neurosurgical procedure, so that the team performing the operation could remove a brain tumour without damaging the motor cortex.
It’s also worth discussing what’s been called the ‘hermonculus’. The homunculus is a composite of localisation data that Penfield obtained from the presurgical evaluation of some 400 patients. Yet, while the homunculus clearly shows the cortical representation of male genitalia, female anatomical parts are conspicuously absent. The reasons for this are unclear. It may be because Penfield worked at a time when it was considered inappropriate to ask about or report certain sensations experienced by his female patients; because female patients felt embarrassed reporting genital sensations to male authority figures; or because Hortense Cantlie, the medical illustrator who drew the homunculus, may have been uncomfortable incorporating female genitalia into her illustrations.
Another possibility is that Penfield simply did not have enough data – just nine of the patients on which the homunculus is based were confirmed as female, only one of whom reported any genital sensations during presurgical evaluation. This was a 27-year-old woman referred to as ‘EC’, who had a tumour removed from her right sensory cortex. Before her surgery, the tumour caused spontaneous seizures that produced a tingling sensation that shifted between her left buttock, labium and breast, and, on the operating table, electrical stimulation of the sensory cortex produced a sensation in her left buttock and a twitching of her left foot.
Penfield and his colleagues thus assumed that the female genitals and breasts are represented in the same region as the male genitalia: adjacent to the representation of the foot, on the inner wall of the cortex, deep inside the longitudinal fissure separating the left and right hemispheres.
We need further investigation into the hermunculus, and to fill in the rest of the female map
We still know very little about the neural representation of the female body. In Penfield’s time, there was only one other case study hinting at how the female genitalia map onto the cortex, that of an epileptic woman diagnosed with ‘erotomania’ (nymphomania) because she experienced vaginal sensations during her seizures; removal of the tumour causing the seizures relieved the patient of those symptoms.
Between then and 2011, there were only 10 other studies investigating the somatotopic organisation of female anatomical parts. These provided conflicting results, hinting at alternative locations for females: some scientists mapped sensations related to female anatomy onto the inner wall of the cortex, consistent with Penfield, but others mapped them further up, at the brain’s apex. Among some researchers, the call is on to resolve the matter with further, active investigation into the hermunculus, and to fill in the rest of the female map. ‘What happens to bodily sensation during pregnancy, menopause … or after surgeries such as oophorectomy[?]’ the neuroscientist Paula Di Noto and colleagues asked in the journal Cerebral Cortex in 2012.
In the most recent such study to address the issue, published in 2022, Andrea Knop of Charité–Universitätsmedizin Berlin and colleagues used functional magnetic resonance (fMRI) to scan 20 women’s brains while stimulation was applied to their clitorises with an air-controlled vibrating membrane placed over disposable underwear just below the pubic mound, to show that the representation of the clitoris in the brain lies adjacent to that of the hips and upper legs, results that ‘provide independent confirmation for the revision of the original homunculus’.
Furthermore, the researchers found that the frequency of sexual intercourse within the 12 months prior to the scan was linked to the thickness of that particular area of the sensory cortex, with the more sexually active participants exhibiting thicker tissue. By contrast, the phase of the menstrual cycle was not associated with differences in thickness of the ‘genital field’.
The sensory and motor strips of the cortex work together to control and coordinate limb movements. The sensory cortex contains cells that process touch and pain information, and the motor cortex contains cells that execute movements by sending signals down the spinal cord to ‘secondary’ cells that activate specific muscles.
But both regions also contain neurons that exhibit properties associated with spatial navigation. These navigation cells, called ‘place cells’, are located in a deep brain structure called the hippocampus. They were first identified in the 1970s in experiments performed on rats, which showed that individual place cells are activated only when the animal enters a specific place in its environment. Since then, researchers have discovered several other navigational cells in and around the hippocampus: head direction cells, which fire when the animal is moving in a specific direction, and grid cells, which fire periodically as the animal moves through an open space.
Two monkeys navigated a small room in a wheelchair controlled by a brain-machine interface to get food
These cells make up the brain’s global positioning system, working together to generate maps of the environment and contributing to formation of the spatial memories we use to find our way around. Recently, two groups of researchers have independently shown that this same spatial navigation system is also found in the brain’s sensory and motor regions.
In a study published in 2018, researchers at Duke University in North Carolina trained two rhesus monkeys to navigate a small room in a wheelchair controlled by a brain-machine interface in order to get food, while recording the activity of hundreds of cells with microelectrode arrays implanted into the animals’ sensory and motor cortices. Unexpectedly, they found that significant numbers of them exhibited place cell-like activity, firing only when the wheelchair was moved into a specific location.
These findings were confirmed in a 2021 study by researchers at Xinqiao Hospital in China, who recorded from the sensory cortex in foraging rats and identified neurons with the properties of place cells, grid cells and head-direction cells.
Although unexpected, the discovery of navigational cells in the sensory and motor cortices is not entirely surprising. Whereas in the hippocampus they function to generate maps and aid navigation, here they are likely to encode the position and orientation of the body within its surroundings.
The discovery of navigational cells in the sensory and motor cortices allows us to expand our thinking about the function of these parts of the brain. Research into the somatotopic organisation of the female body suggests that the homunculus needs to be updated. At the same time, a team of researchers at the Washington University School of Medicine in St Louis is now arguing that the homunculus is entirely wrong and needs to be completely redrawn.
Evan Gordon, Nico Dosenbach and colleagues set out to replicate Penfield’s findings by using fMRI to scan the brains of seven volunteers at rest and as they performed various movement tasks, generating high-resolution brain maps for each. They then verified their results with data from three large, publicly available datasets, which between them contain brain-scanning data collected from some 50,000 people.
They found that movement of the feet, hands and face was associated with the parts of the motor cortex identified by Penfield, but that interspersed between these discrete regions were other areas that did not seem to be involved in movement at all. These other regions were thinner than the flanking regions associated with individual parts of the body, and were connected to each other, both within the same and between the two hemispheres of the brain, to form a chain running down the motor strip.
They argue that Penfield’s classic homunculus is wrong or at least spectacularly incomplete
Further investigation revealed that these areas are also strongly connected to distant brain regions involved in ‘executive’ functions such as thinking and planning, visual processing and the processing of touch, pain and internal bodily signals, and that they became active when the participants thought about moving.
The researchers propose that these areas form a network that integrates whole-body movements and anticipates them with appropriate changes in arousal, posture, breathing and heart function.
‘All of these connections make sense if you think about what the brain is really for,’ Dosenbach said in an interview. ‘The brain is for successfully behaving in the environment so you can achieve your goals without hurting or killing yourself. You move your body for a reason. Of course, the motor areas must be connected to executive function and control of basic bodily processes, like blood pressure and pain.’
In light of their findings, Gordon, Dosenbach and colleagues argue that Penfield’s classic homunculus is wrong or at least spectacularly incomplete, and needs to be radically revised to include the network they identified, which they have named the somato-cognitive action network (SCAN).
‘Penfield was brilliant, and his ideas have been dominant for 90 years … [but] once we started looking, we found lots of published data that didn’t quite jibe with his ideas, and alternative interpretations that had been ignored,’ Dosenbach said. ‘We pulled together a lot of different data in addition to our own observations, and zoomed out and synthesised it, and came up with a new way of thinking about how the body and the mind are tied together.’
What does this mean for neurosurgeons who use the homunculus to guide their scalpel? Performing surgery for epilepsy is extremely challenging due to the high risk of damaging the sensory or motor strips. Typically, the motor cortex generates seizures that are limited to certain parts of the body, but may spread to adjacent parts, and the non-movement regions identified by Gordon, Dosenbach and colleagues could, in theory, generate seizures that spread in unusual ways.
‘The likelihood that a seizure remains in this area without spreading to adjacent motor areas seems low, and I would expect typical [symptoms] in most situations,’ David Steven, professor of neurosurgery at Western University in London, Ontario, told me. With the brain regions intermingled, the surgery could be high risk, except in ‘the face area, which is usually safe as there is representation [on both sides of the brain].’
In practical terms, the little man in the brain still looms large. ‘For pre-surgical work-up and intra-operative decisions, it remains critical and very relevant,’ says Steven. ‘It may be oversimplified but, practically speaking, it remains essential.’
Mapping in finer detail will allow for prostheses that provide more realistic sensory feedback
Beyond the operating table, knowledge of how the body maps onto the motor cortex has been instrumental in the development of brain-machine interfaces that control prostheses to restore function to paralysed patients and amputees. These devices typically consist of a microelectrode array implanted into the motor cortex, which reads the brain activity associated with planning and executing movements and translates it into commands that can be used to control a wheelchair or robotic arm.
Early versions of these prostheses were cumbersome, but they are becoming more sophisticated by the day, and some of the newer devices can simultaneously stimulate the sensory cortex to provide sensory feedback. As well as restoring some sense of touch, this gives the user better control over the device, and can also reduce phantom limb pain that most amputees feel. Mapping the sensory homunculus in even finer detail will undoubtedly allow for prostheses that provide increasingly realistic sensory feedback to the user.
In the not too distant future, this knowledge, combined with a better understanding of brain activity underlying different types of touch, could also be used to develop the next generation of ‘haptic devices’, consisting of headsets that can precisely target the sensory cortex with small electrical or magnetic pulses to elicit realistic sensations of various kinds in any part of the user’s body.
From the future of artificial limbs to the future of gaming, the little man (and woman) in the brain may just be getting started – even if we’re still learning how they operate, in full.