The French mathematician Pierre-Simon Laplace (1749-1827) believed that the Universe was a piece of machinery, and that physics determines everything. Napoleon, who had read up on Laplace’s work, confronted him about the conspicuous absence of a creator in his theory. ‘I had no need of that hypothesis,’ came the reply. Laplace might have said the same thing about free will, which his mechanistic universe rendered superfluous.
Since Laplace’s day, scientists, philosophers and even neuroscientists have followed his lead in denying the possibility of free will. This reflects a widespread belief among theoretical physicists that if you know the initial values of the variables that characterise a physical system, together with the equations that explain how these variables change over time, then you can calculate the state of the system at all later times. For example, if you know the positions and velocities of all the particles that make up a gas in a container, you can determine the positions and velocities of all those particles at all later times. This means that there should be no freedom for any deviation from this physically determined trajectory.
Consider, then, that everything we see around us – rocks and planets, frogs and trees, your body and brain – is made up of nothing but protons, electrons and neutrons put together in very complex ways. In the case of your body, they make many kinds of cells; in turn, these cells make tissues, such as muscle and skin; these tissues make systems, such as the heart, lungs and brain; and these systems make the body as a whole. It might seem that everything that’s happening at the higher, ‘emergent’ levels should be uniquely determined by the physics operating beneath them. This would mean that the thoughts you’re having at this very moment were predetermined at the start of the Universe, based on the values of the particle physics variables at that time.
Now you might be forgiven for doubting whether William Shakespeare’s sonnets, Winston Churchill’s speeches and the words in Stephen Hawking’s book A Brief History of Time (1988) really came into being in this way. And you would be right to doubt: there are many problems with the skeptics’ position.
At very small scales, quantum theory underlies what’s happening in the world. Heisenberg’s uncertainty principle introduces an unavoidable fuzziness and an irreducible uncertainty in quantum outcomes. You might know the value of one variable, such as a particle’s momentum, but that means you can’t accurately detect another, such as its position. This seems to fundamentally undermine the allegedly iron-clad link between initial data and physical results. However, this is controversial, so I’ll set it aside for now, as important as it is. Instead, I’ll focus on key aspects of causation that occur in the molecular biology of neurons in the brain.
One of the most astounding discoveries of the previous century was that biological activity at the micro level is literally grounded in the physical shape of biological molecules, particularly DNA, RNA and proteins. This discovery became possible only when X-ray crystallography had progressed to the point of allowing us to determine the extraordinarily complex detailed structure and foldings of these molecules.
The structure of these molecules is truly the secret of life, as Francis Crick and James Watson exclaimed when they discovered the double helix structure of DNA, helped by the work of Rosalind Franklin. This deservedly led to huge public excitement about how DNA molecules encode our genetic inheritance. However, it is the structure of other molecules – proteins and associated messenger molecules – that in fact makes things happen at the cellular level. DNA is important only because it codes for the proteins that do the real biological work. For example, haemoglobin in blood cells transports oxygen from the lungs to the rest of the body. Rhodopsin in the eye absorbs light and turns it into electrical signals. Kinesin and dynein are motor proteins that transport materials from one place to another in a cell. Enzymes speed up chemical reactions by such huge amounts that they essentially turn them on and off. Voltage-gated ion channels serve as biological versions of transistors, while ligand-gated ion channels allow messenger molecules (‘ligands’) such as neurotransmitters to convey information from one cell to another in the brain. And so it goes. And all this functioning follows from the details of the complex shapes of these proteins.
This means that, to link physics and biology, we need to look at the theory that underlies molecular shape. And that theory is quantum chemistry, based in the fundamental equation of quantum physics: the Schrödinger equation. In quantum theory, the state of a system is described by what’s known as its wave function, which determines the probabilities of different outcomes when events take place. The Schrödinger equation governs how the wave function changes with time. For example, it governs the process of quantum tunnelling, which in turn underlies important physical effects such as how the Sun generates energy via nuclear fusion, photosynthesis in plants, and flash memories you use to store data in computer USB flash drives.
I will take for granted the validity of the Schrödinger equation, which is one of the best-tested equations in physics. To link this to the functioning of life, we need to apply the Schrödinger equation to the wave function of the relevant molecules – in this case, proteins – so as to determine how their shape will change with time. So the actual question is: does the Schrödinger equation, together with the initial state of the wave function describing everything that existed in the early Universe, determine everything I think today because it determines the states of all the biomolecules in my body?
The confounding thing for free-will skeptics is that all outcomes don’t depend only on the equations and the initial data. They also depend on constraints. An example is an apple under the influence of gravity, such as the one that Isaac Newton watched fall to the ground from a tree at Woolsthorpe Manor. That was its unconstrained motion.
Now suppose Newton had suspended the apple from a branch of the tree by a string attached to its stalk. It would thereby have been turned into a pendulum, because the string constrained its motion. Instead of dropping to the ground, it would have swung back and forth in a circular arc under the branch, with its state of motion determined uniquely by its initial position and velocity. Consequently, the motions of all the billions of atoms that make up the apple would then also be determined by the string. It would make each of them also move in a circular arc under the support. This is how constraints shape outcomes.
Now let’s examine variation of the constraint over time. While the apple was swinging peacefully back and forth, imagine that Newton cut the string. The apple would then have fallen to the ground. The initial state (its speed in a circular arc when it started) no longer determined the outcome. It was the unexpected cutting of the string that determined what happened, because it removed the previous constraint. The moral of the story is that, when constraints vary, outcomes are not determined by initial conditions; they depend on the way that the constraints change with time.
In the case of the biomolecules that underlie the existence of life, it’s the shape of the molecule that acts as a constraint on what happens. These molecules are quite flexible, bending around joints rather like hinges. The distances between the atomic nuclei in the molecules determine what bending is possible. Any particular such molecular ‘conformation’ (a specific state of folding) constrains the motions of ions and electrons at the underlying physical level. This can happen in a time-dependent fashion, according to biological needs. In this way, biology can reach down to shape physical outcomes. It changes constraints in the applicable Schrödinger equation.
It’s a biochemical version of a door that opens only if the key is the right shape for the specific lock
A key case are the ion channels in cell walls that separate the interior of a cell from its exterior. Ions are atoms that have become electrically charged because they have lost or gained an electron. Sodium and potassium atoms are positively charged because they have lost an electron, while chlorine ions are negatively charged because they have gained an electron. Ion channels are proteins imbedded in the cell wall, controlling the flow of ions in and out of the cell. They can be open or closed, depending on the position of their hinged parts. They thereby either allow movement of ions into or out of the cell (depending on their type), or prevent it.
This gating plays a crucial role in brain functioning. For example, neurons are linked to each other by fibres called axons. Voltage-gated ion channels in the axon wall open or close based on the difference in electrical voltage between the inside and the outside of the cell. The ions moving in and out of axons through these ion channels cause an electrical signal to move along the neuron fibres, thus creating the electric nerve impulses by which we think (called ‘spike chains’, because they consist of a series of little voltage surges or ‘spikes’). These ion channels are a biological analogue of transistors in computers, which allow currents to flow or not, based in the voltage difference between two parts of the circuit.
Another type of ion channel is a ligand-gated one, which occurs in the synapses that connect neurons to each other. The process is a complex biochemical version of opening a door with a key: it will open only if the key is the right shape for the specific lock. A ligand, in this case a neurotransmitter, is a messenger molecule that binds to a receptor on the ion channel, and so opens it by changing its shape.
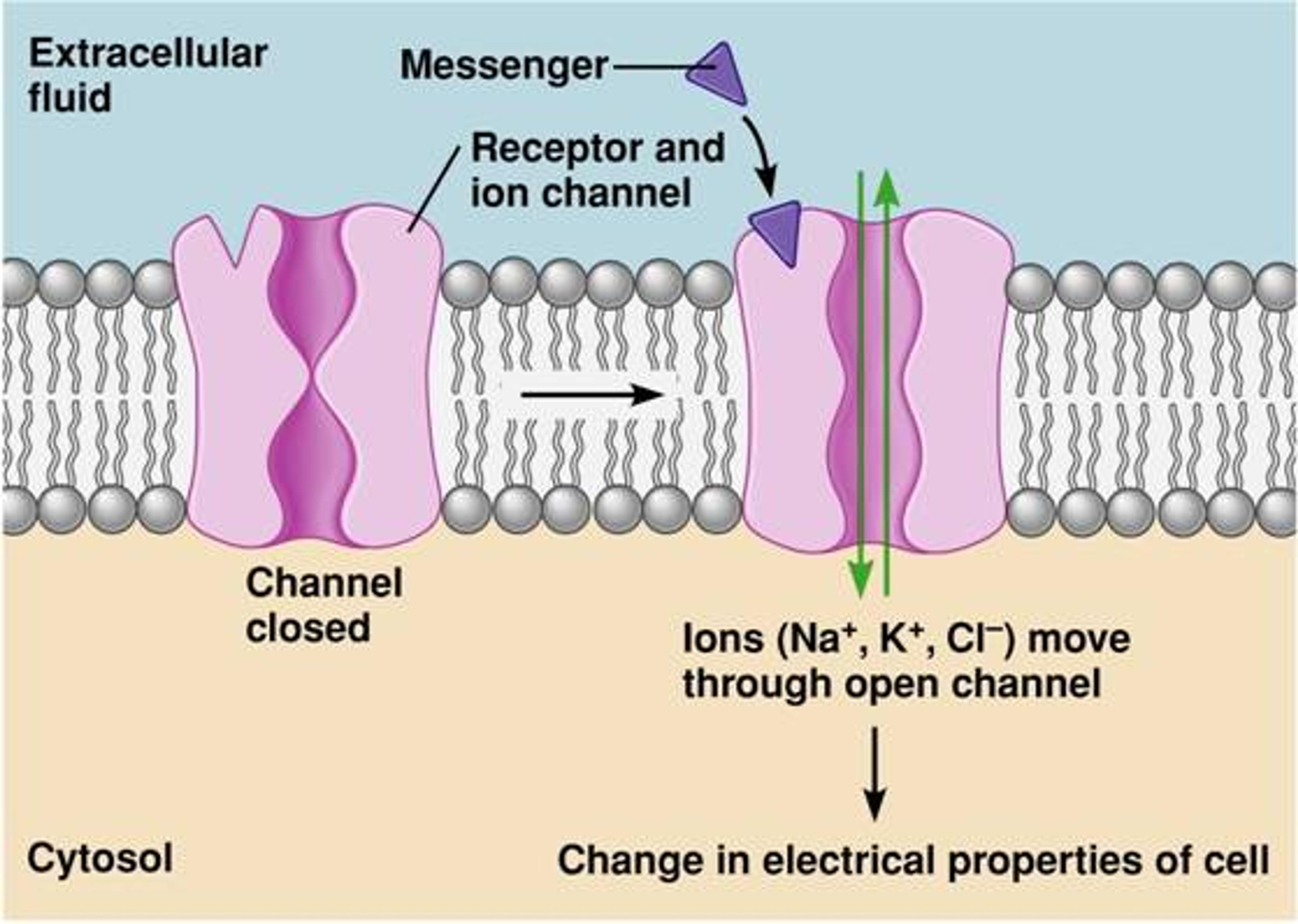
Figure 1: Ligand-gated ion channel function. This is how messenger molecules control physical outcomes inside the cell. From Membrane Receptors
This allows ions to flow into a neuron, changing a chemical to an electrical signal. The ion channel allows ions to enter a cell only when a ligand is bound to a receptor and changes the shape of the ion channel so that it opens. If no ligand is present, the channel is closed and no ions can enter. The binding that causes this opening and closing is a consequence of the particular shape of the ligand: it can bind if and only if it has precisely the right shape for that specific receptor. The gating function, then, is a consequence of the detailed shape of the ion channel.
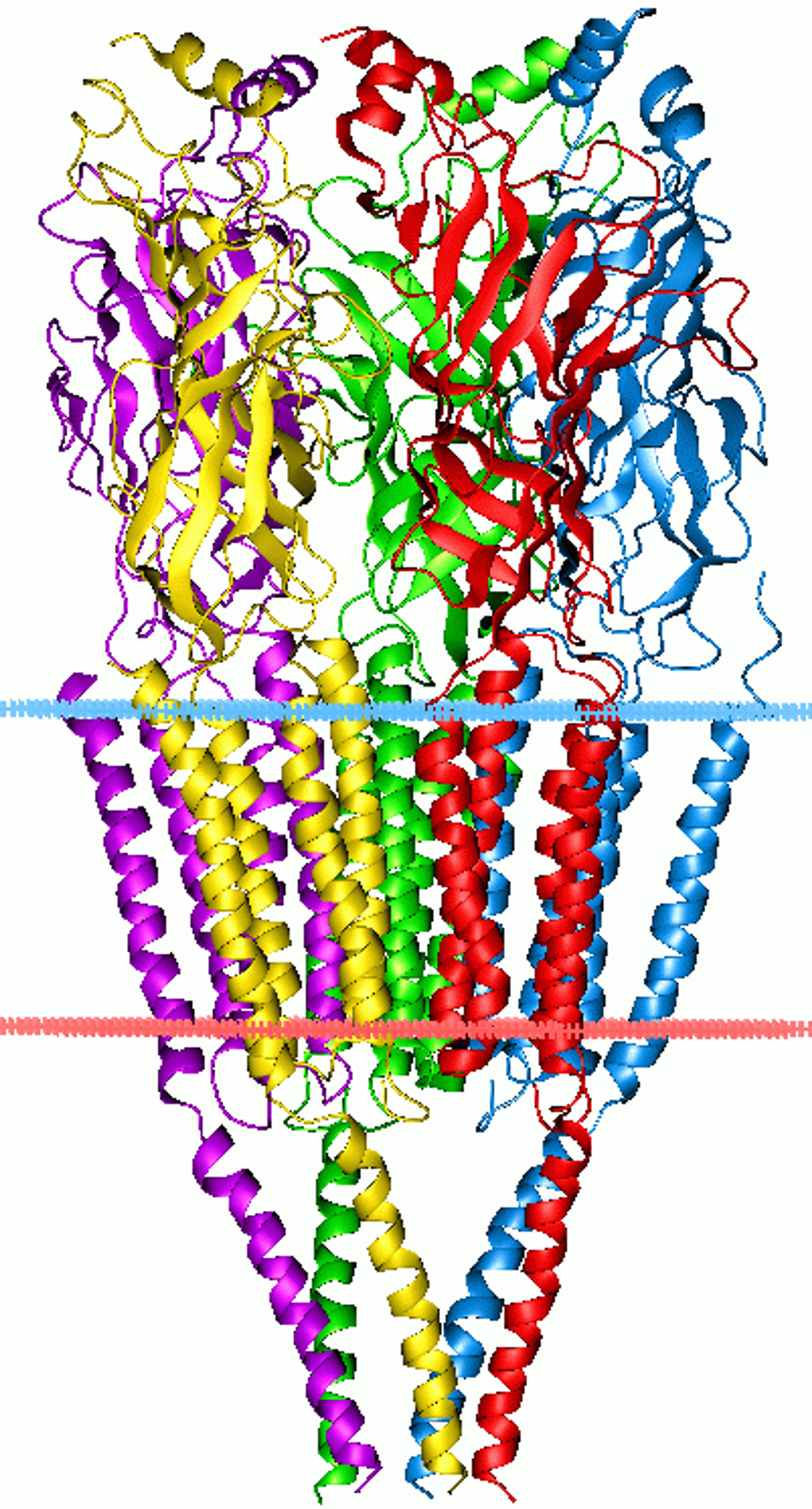
Figure 2: Ligand-gated ion channel. It is such detailed molecular structures that are the secret of life. Courtesy Wikipedia
This is how signalling molecules alter constraints on the flow of ions through ion channels in cell walls. The constraints are different before and after the binding of a messenger molecule. This time-dependent nature of binding controls ion flows at the synapses where one neuron joins to another one. It is these changes of molecular shape, rather than initial conditions, that determine which specific solutions of the molecular Schrödinger equation will occur in your brain when you’re thinking. They underlie the possibility of thought.
So what determines which messages are conveyed to your synapses by signalling molecules? They are signals determined by thinking processes that can’t be described at any lower level because they involve concepts, cognition and emotions in an essential way. Psychological experiences drive what happens. Your thoughts and feelings reach ‘down’ to shape lower-level processes in the brain by altering the constraints on ion and electron flows in a way that changes with time.
For example, suppose you’re walking down the street, and just in front of you a terrible accident happens – smashed-up cars, people injured, blood everywhere. You react with horror: sympathy for those who’ve been hurt, fear that they will die, a guilty sense of relief that it didn’t happen to you. These are all mental events that take place because of the way your brain functions at the psychological level, based on some combination of past experience and innate responses. None of those qualities – sympathy, fear, guilt – occur at the ion or synapse level. These high-level mental operations act down to alter the shape of ion channels, and so change the motions of billions of ions and electrons in your brain. In an intricate causal dance between levels in your brain, those thoughts are able to occur because of the underlying spike chains, but it’s their essentially psychological nature – what it means to recognise an accident, which thoughts flow through your mind as you decide what to do, what it feels like to experience the shock of seeing the event – that causes what happens. Physics enabled what took place in your head and body, but didn’t determine it; your mental interpretation of the event did.
Learning and memory offer another example of how downward causal effect shapes the underlying physics. Memory is controlled by genes switching on and off, known as ‘gene regulation’. Genes, made of DNA molecules, contain the information needed for cells to manufacture specific proteins at the right place and time. Now there’s a problem here: nearly every cell in our body has the same genes, and codes for all the proteins that occur in the rest of the body. But each cell needs specific proteins to be produced, depending on context. So identical DNA strands produce different proteins in different cells at various times. How does this happen? It occurs by gene regulation: genes being switched on and off, and so being expressed or not. Gene regulation is controlled by proteins called transcription factors. These are regulatory proteins that bind to specific sites on a DNA molecule that recognise their shape, and so determine which proteins will be produced when the DNA is read.
This process of gene regulation strengthens or weakens neural connections in our brain. The cortex has columns of densely packed neurons, connected to each other by an immensely complex network of connections. The broad structure of that network is the same for everyone, but the details are not. It’s the detailed structure in each brain that embodies the differences between us, and specifically our long-term memories. The details of which neuron is connected to which, and the strength of each connection, have been shaped by our own history and memories.
Learning changes structure at the macro scale, which reaches down to alter micro connections
How does any of this happen? As the Austrian-American doctor Eric Kandel explained in his Nobel Prize Lecture from 2000, the process of learning at the mental level leads to changed patterns of gene expression, and so specific proteins being produced, which alter the strengths of neural connections at synapses. This changes the strength of connections between neurons, thereby storing memories.
Such learning is a psychological happening. You might remember your pleasure on eating a delicious meal, the details of a Yo-Yo Ma rendition of a Bach sonata, or the painful memory of the car crash. Once again, these are irreducible psychological events: they can’t be described at any lower level. They reach down to alter neuronal connections over time. These changes can’t be predicted on the basis of the initial state of the neural connections (your neurons did not know that the car crash was about to happen) – but, afterwards, they constrain electron flows differently, because connections have changed. Learning changes structure at the macro scale (we have a ‘plastic brain’), which reaches down to alter micro connections and the details of electron flows at the bottom.
Another case in point is rational thought. In about 500 BCE, the Pythagoreans discovered something startling: that the square root of two is irrational. That is, if 2 is the product of a number N with itself (N times N is 2), then N can’t be expressed as a ratio of whole numbers, for example N cannot be 3/2. This was so shocking that the discovery was treated as an official secret. It wasn’t something they wished to be true, and it was dangerous to know it.
This scandalous conclusion was reached by rational argument according to mathematical rules – rules that shaped the processes that took place in the brain of Hippasus of Metapontum. Abstract logic was driving those brain operations at all levels from the psychological (‘What ratio of integral numbers gives me the square root of 2?’) to electrical impulses flying along nerve fibres, to the ions moving in and out of those fibres through ion channels, to the interactions between electrons and protons that enabled the whole thing to happen.
We don’t know the details of how thoughts are encoded by spike chains, but we do know this coding must occur. The spike trains must somehow reflect the structure of the logical argument that the square root of 2 is irrational. If we memorise this proof, the mathematical argument reaches down to reshape neuronal connections via gene regulation as the memory is stored. The logical argument is then literally embodied in details of brain connections.
What these instances show is that psychological understandings reach down to shape the motions of ions and electrons by altering constraints at the physics-level over time. That is, mental states change the shape of proteins because the brain has real logical powers. This downward causation trumps the power of initial conditions. Logical implications determine the outcomes at the macro level in our thoughts, and at the micro level in terms of flows of electrons and ions.
These examples are of course contentious because they involve the human brain and thought, and implicitly the issue of free will. However, the analogous issue also arises in the case of computer algorithms. An algorithm working out answers to specific questions – What pages will Google display to you? How much can you withdraw with this credit card? Can I book the 8:45am train tomorrow? – is again based in abstract logic. This logic is explicitly coded in a computer program written in a high-level programming language such as FORTRAN, Java or Python.
The equations at the foundations of physics have no direct relation to such algorithms. But computer programs are clearly causally effective: they alter outcomes in the world. Programs determine what happens according to the logic of the algorithm acting on the relevant data. This is what computer science students study. That logic cascades down to the digital level, where it determines what happens to electron flows by altering applied voltages that change the structure of electron conduction channels in transistors. Before the program is loaded into the computer, the relevant algorithm is not represented in its innards; after the program is loaded, it is. Constraints at the macro level have been changed by loading the program. The physics dances to the tune of the algorithm, as voltages at the micro level change microsecond by microsecond during the program execution.
Free-will skeptics ignore the kind of time-dependent constraints that I discuss here, which enable downwards causation in biology in general and brain function in particular. Of course, nothing about molecular biology contradicts the physics that underlies all material existence. Rather, it provides an extraordinarily complex context where things work out according to that context. Even though our brains are indeed made up of fundamental particles, high-level function emerges through the interaction of upward and downward causal processes.
There’s a major element of randomness in what happens in cosmology and in molecular biology
But still a nagging thought occurs: if the initial data were known for the entire Universe, then why can’t it determine all these lower-level dynamics in a mechanistic way? After all, aren’t they just smallscale details in this larger picture, where one can claim that no constraints occur? The Universe is by definition all that there is, so it can’t be constrained by effects from a larger environment. Might physics not be deterministic in that case, and my argument fall apart?
The response is twofold. First, there’s a major element of randomness in what happens both in cosmology at large scales and in molecular biology at small scales. At the large scales, in cosmology we have difficulty in getting the details of what happens right, even at galactic scales; we work only with statistical likelihoods. There are significant unsolved problems, such as how dark matter clumps around galaxies at scales hugely greater than that of the solar system. We can’t realistically determine from studies based in the initial conditions in cosmology what happens at smaller scales such as that of the Sun or Earth. There’s no hope whatsoever of predicting details down to the scale of the human body.
However, skeptics still say that it’s just a matter of lacking enough data and computing power. In principle, it could work. But actually, it won’t, because of what happens at the microscale. At molecular scales, the processes at work forget initial data due to billions on billions of collisions between molecules every second. Biology thrives on that disorder – a ‘molecular storm’, as Peter Hoffman calls it in his book Life’s Ratchet (2012). Molecular machines do work, such as kinesin moving cargos from one place to another in the cell, extracting order out of the chaos. Far from physics having the determinate nature envisaged by Laplace, all the molecules’ argy-bargy every microsecond means that the details of their initial state of motion are irretrievably lost. It’s this molecular-level chaotic motion that prevents micro-determinism in practice.
But how can order emerge out of this chaos? As explained by Denis Noble and Raymond Noble in their paper for the journal Chaos in 2018, molecular randomness gives cellular mechanisms the option of choosing the outcomes they want, and discarding those they don’t. This power of choice enables physiological systems such as the heart and brain to function in a way that isn’t enslaved by the lower-level interactions, but rather choosing the outcomes of the preferred interactions from a multitude of options. In this way, a layer of order can emerge from the disorder – and micro data – at the lower level. This isn’t conclusive proof that free will exists, but at least it opens up a way for it to exist.
For the sake of argument, let’s suppose I’m wrong. Let’s ignore all these issues and take the deterministic view seriously. It implies that the words of every book ever written – the Encyclopaedia Britannica, Das Kapital, the Harry Potter series – were encoded into the initial state of the Universe, whatever that was. No logical thinking by a human played a causal role in the specific words of these books: they were determined by physics alone.
It’s unclear how any words could have been encoded into the Universe, which led to apparently random fluctuations at the time when matter and radiation decoupled from each other. How would they have been represented in those fluctuations? It’s virtually impossible that they could have affected the detailed brain-state of the authors when they wrote their books. The issue of quantum uncertainty adds another layer of implausibility to these claims. But let’s set all these major issues aside for now. Let’s suppose it is indeed possible that present-day brain-states are determined by initial conditions in the Universe, because causally deterministic physics underlies all.
The problem then is, how did all those words get there? Was there a demiurge who coded all that stuff into the detailed initial state of the Universe? It’s certainly not there in the Schrödinger equation per se, or in a randomly determined set of fluctuations in the early Universe as is normally envisaged in cosmological studies. By definition, they don’t encode either any detailed information or any logical argumentation.
So how could that data have got there? Not just for one book, but for all the books ever written? Is that really a believable story, or some kind of creationist myth?
Genuine mental functioning and the ability to make decisions in a rational way is a far more persuasive explanation of how books get written. That this is possible is due to the extraordinary hierarchical structure of our brain and its functioning. And that functioning is enabled by downward causation from the psychological to the physical levels, with outcomes at the physics level determined by constraints that change over time. No violation of physical laws need occur.
Physics has made huge strides since the days of Laplace; indeed, it would be completely unrecognisable to him. Yet there are still physicists today who confidently proclaim that we can’t have free will because physics determines everything, including brain functioning – entirely ignoring the complex context and the power of constraints.
If you seriously believe that fundamental forces leave no space for free will, then it’s impossible for us to genuinely make choices as moral beings. We wouldn’t be accountable in any meaningful way for our reactions to global climate change, child trafficking or viral pandemics. The underlying physics would in reality be governing our behaviour, and responsibility wouldn’t enter into the picture.
That’s a devastating conclusion. We can be grateful it’s not true.