One of the most radical and important ideas in the history of physics came from an unknown graduate student who wrote only one paper, got into arguments with physicists across the Atlantic as well as his own advisor, and left academia after graduating without even applying for a job as a professor. Hugh Everett’s story is one of many fascinating tales that add up to the astonishing history of quantum mechanics, the most fundamental physical theory we know of.
Everett’s work happened at Princeton in the 1950s, under the mentorship of John Archibald Wheeler, who in turn had been mentored by Niels Bohr, the godfather of quantum mechanics. More than 20 years earlier, Bohr and his compatriots had established what came to be called the ‘Copenhagen Interpretation’ of quantum theory. It was never a satisfying set of ideas, but Bohr’s personal charisma and the desire on the part of scientists to get on with the fun of understanding atoms and particles quickly established Copenhagen as the only way for right-thinking physicists to understand quantum theory.
In the Copenhagen view, we distinguish between microscopic quantum systems and macroscopic observers. Quantum systems exist in superpositions of different possible measurement outcomes, called ‘wave functions’. A spinning electron, for example, has a wave function describing a superposition of ‘spin-up’ and ‘spin-down’. It’s not merely that we don’t know the spin of the electron, but that the value of the spin does not exist until it is measured. An observer, by contrast, obeys all the rules of familiar classical physics. At the moment that an observer measures a quantum system, that system’s wave function suddenly and unpredictably collapses, revealing some definite spin or whatever has been measured.
There are apparently, therefore, two completely different ways in which quantum systems evolve. When we’re not looking at them, wave functions change smoothly according to the Schrödinger equation, written down by Erwin Schrödinger in 1926. But when we do look at them, wave functions act in a totally different way, collapsing onto some particular outcome.
If this seems unsatisfying, you’re not alone. What exactly counts as a measurement? And what makes observers so special? If I’m made up of atoms that obey the rules of quantum mechanics, shouldn’t I obey the rules of quantum mechanics myself? Nevertheless, the Copenhagen approach became enshrined as conventional wisdom, and by the 1950s it was considered somewhat ill-mannered to question it.
That didn’t bother Everett. The seeds of his visionary idea, now known as the Many-Worlds formulation of quantum mechanics, can be traced to a late-night discussion in 1954 with fellow young physicists Charles Misner (also a student of Wheeler’s) and Aage Peterson (an assistant of Bohr’s, visiting from Copenhagen). All parties agree that copious amounts of sherry were consumed on the occasion.
Under Wheeler’s guidance, Everett had begun thinking about quantum cosmology: the study of the entire Universe as a quantum system. Clearly, he reasoned, if we’re going to talk about the Universe in quantum terms, we can’t carve out a separate classical realm. Every part of the Universe will have to be treated according to the rules of quantum mechanics, including the observers within it. There will be only a single quantum state, described by what Everett called the ‘universal wave function’.
If everything is quantum, and the Universe is described by a single wave function, how is measurement supposed to occur? It must be, Everett reasoned, when one part of the Universe interacts with another part of the Universe in some appropriate way. That is something that’s going to happen automatically, he noticed, simply due to the evolution of the universal wave function according to the Schrödinger equation. We don’t need to invoke any special rules for measurement at all; things bump into each other all the time.
Imagine that we have a spinning electron in some superposition of up and down. We also have a measuring apparatus, which according to Everett is a quantum system in its own right. Imagine that it can be in superpositions of three different possibilities: it can have measured the spin to be up, it can have measured the spin to be down, or it might not yet have measured the spin at all, which we call the ‘ready’ state.
The world has ‘branched’ into a superposition of these two possibilities
The fact that the measurement apparatus does its job tells us how the quantum state of the combined spin + apparatus system evolves according to the Schrödinger equation. Namely, if we start with the apparatus in its ready state and the electron in a purely spin-up state, we are guaranteed that the apparatus evolves to a pure measured-up state, like so:
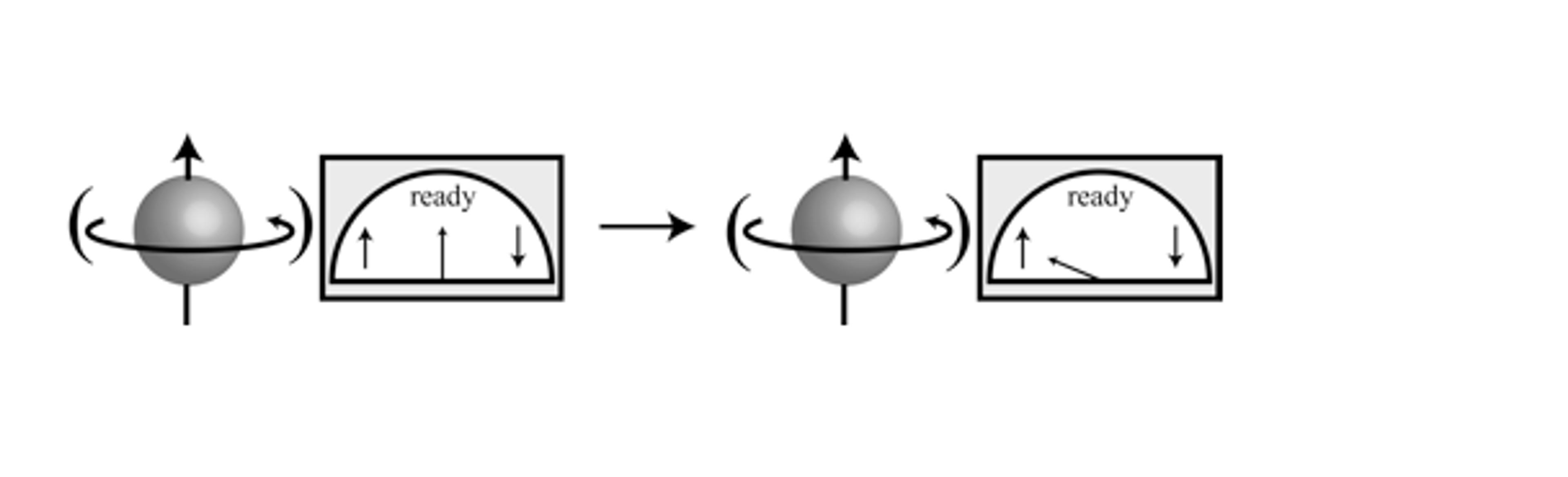
The initial state on the left can be read as ‘the electron is in the up state, and the apparatus is in its ready state’, while the one on the right, where the pointer indicates the up arrow, is ‘the electron is in the up state, and the apparatus has measured it to be up’.
Likewise, the ability to successfully measure a pure-down spin implies that the apparatus must evolve from ‘ready’ to ‘measured down’:
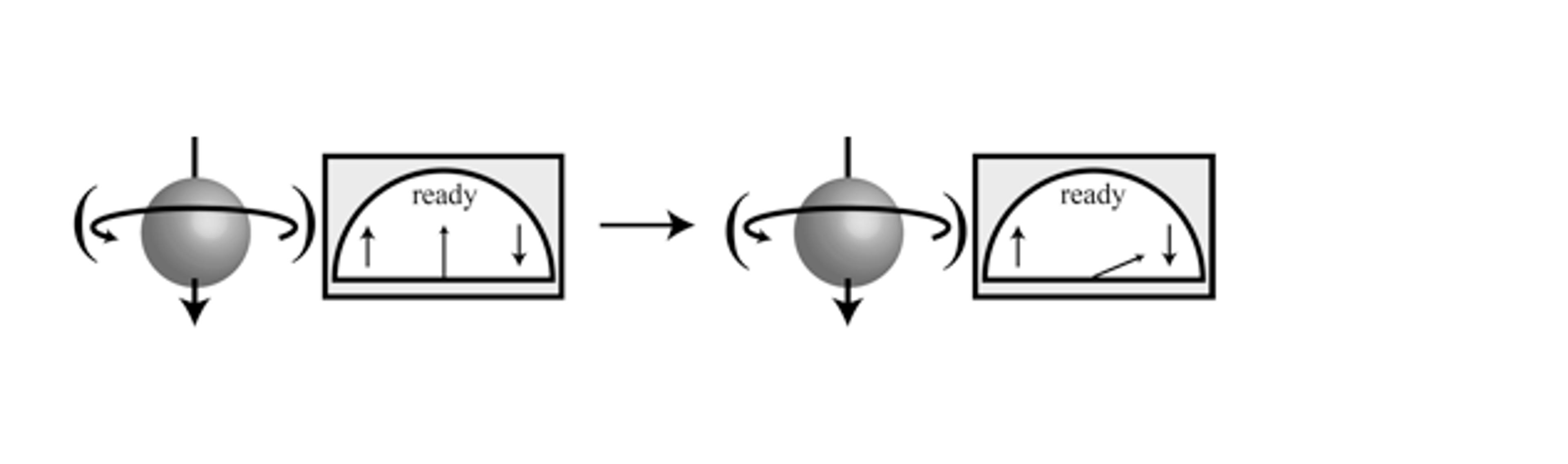
What we want, of course, is to understand what happens when the initial spin is not in a pure up or down state, but in some superposition of both. The good news is that we already know everything we need. The rules of quantum mechanics are clear: if you know how the system evolves starting from two different states, the evolution of a superposition of both those states will just be a superposition of the two evolutions. In other words, starting from a spin in some superposition and the measurement device in its ready state, we have:
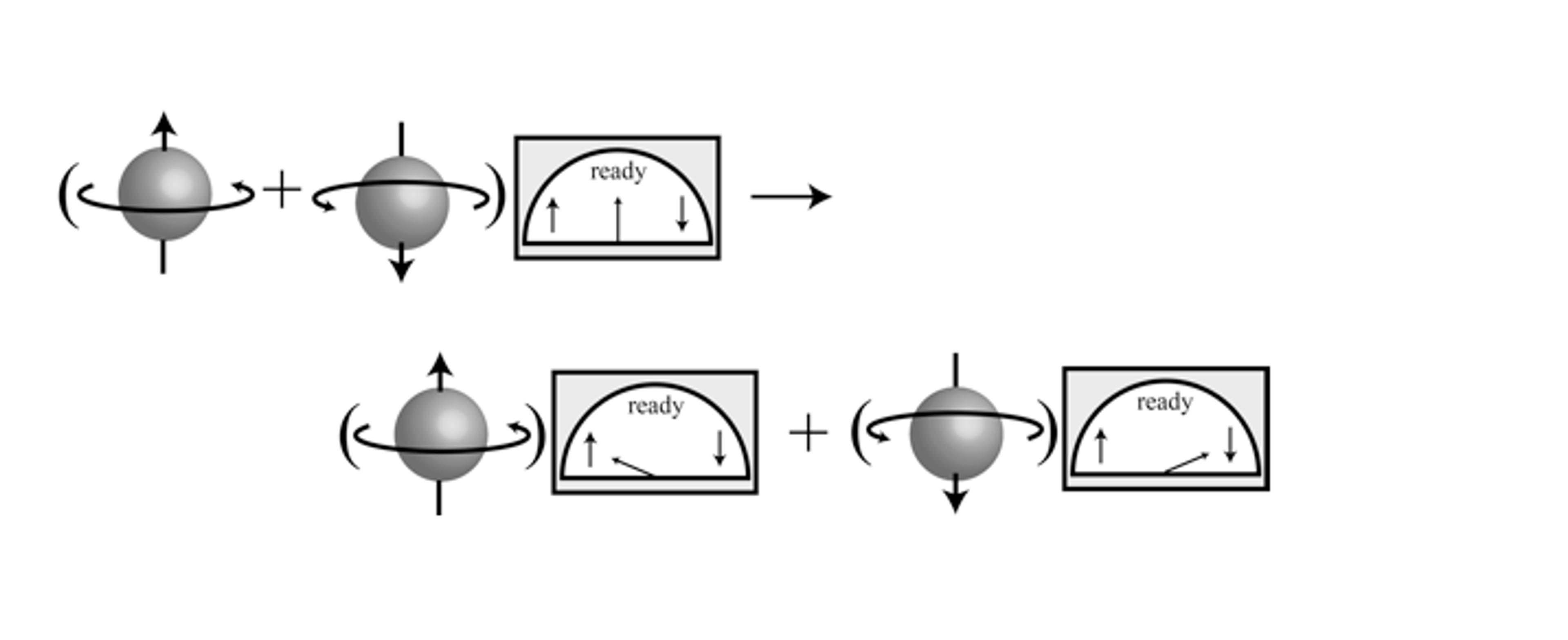
The final state now is an entangled superposition: the spin is up and it was measured to be up, plus the spin is down and it was measured to be down. This is the clear, unambiguous, definitive final wave function for the combined spin + apparatus system, if all we do is evolve it according to the Schrödinger equation. The world has ‘branched’ into a superposition of these two possibilities.
Everett’s insight was as simple as it was brilliant: accept the Schrödinger equation. Both of those parts of the final superposition are actually there. But they can’t interact with each other; what happens in one branch has no effect on what happens in the other. They should be thought of as separate, equally real worlds.
This is the secret to Everettian quantum mechanics. We didn’t put the worlds in; they were always there, and the Schrödinger equation inevitably brings them to life. The problem is that we never seem to come across superpositions involving big macroscopic objects in our experience of the world.
The traditional remedy has been to monkey with the fundamental rules of quantum mechanics in one way or another. The Copenhagen approach is to disallow the treatment of the measurement apparatus as a quantum system in the first place, and to treat wave-function collapse as a separate way the quantum state can evolve. As Everett would later put it: ‘The Copenhagen Interpretation is hopelessly incomplete because of its a priori reliance on classical physics … as well as a philosophic monstrosity with a “reality” concept for the macroscopic world and denial of the same for the microcosm.’
The Many-Worlds formulation of quantum mechanics removes once and for all any mystery about the measurement process and collapse of the wave function. We don’t need special rules about making an observation: all that happens is that the wave function keeps chugging along in accordance with the Schrödinger equation. And there’s nothing special about what constitutes ‘a measurement’ or ‘an observer’ – a measurement is any interaction that causes a quantum system to become entangled with the environment, creating a branching into separate worlds, and an observer is any system that brings about such an interaction. Consciousness, in particular, has nothing to do with it. The ‘observer’ could be an earthworm, a microscope or a rock. There’s not even anything special about macroscopic systems, other than the fact that they can’t help but interact and become entangled with the environment. The price we pay for such a powerful and simple unification of quantum dynamics is a large number of separate worlds.
Everett’s theory was a direct assault on Bohr’s picture, and he enjoyed illustrating this assault in vivid language
Even in theoretical physics, people do sometimes get lucky, hitting upon an important idea more because they were in the right place at the right time than because they were particularly brilliant. That’s not the case with Everett; those who knew him testify uniformly to his incredible intellectual gifts, and it’s clear from his writings that he had a thorough understanding of the implications of his ideas. Were he still alive, he would be perfectly at home in modern discussions of the foundations of quantum mechanics.
What was hard was getting others to appreciate those ideas, and that included his advisor. Wheeler was personally very supportive of Everett, but he was also devoted to his own mentor Bohr, and was convinced of the basic soundness of the Copenhagen approach. He simultaneously wanted Everett’s ideas to get a wide hearing, and to ensure that they weren’t interpreted as a direct assault on Bohr’s way of thinking about quantum mechanics.
Yet Everett’s theory was a direct assault on Bohr’s picture. Everett himself knew it, and enjoyed illustrating the nature of this assault in vivid language. In an early draft of his thesis, he used an analogy of an amoeba dividing to illustrate the branching of the wave function:
[O]ne can imagine an intelligent amoeba with a good memory. As time progresses, the amoeba is constantly splitting, each time the resulting amoebas having the same memories as the parent. Our amoeba hence does not have a life line, but a life tree.
Wheeler was put off by the blatantness of this (quite accurate) metaphor, scribbling in the margin of the manuscript: ‘Split? Better words needed.’ Advisor and student were constantly tussling over the best way to express the new theory, with Wheeler advocating caution and prudence while Everett favoured bold clarity.
In 1956, as Everett was working on finishing his dissertation, Wheeler visited Copenhagen and presented the new scenario to Bohr and his colleagues, including Petersen. He attempted to present it, anyway; by this time, the wave-functions-collapse-and-don’t-ask-embarrassing-questions-about-exactly-how school of quantum theory had hardened into conventional wisdom, and those who accepted it weren’t interested in revisiting the foundations when there was so much interesting applied work to be done. Letters from Wheeler, Everett and Petersen flew back and forth across the Atlantic, continuing when Wheeler returned to Princeton and helped Everett to craft the final form of his dissertation. It omitted many of the juicier sections Everett had originally composed, including examinations of the foundations of probability and information theory, and an overview of the quantum measurement problem, focusing instead on applications to quantum cosmology. (No amoebas appear in the published paper, but Everett did manage to insert the word ‘splitting’ in a footnote added in proof while Wheeler wasn’t looking.)
But Everett decided not to continue the academic fight. Before finishing his PhD, he accepted a job at the Weapons Systems Evaluation Group for the US Department of Defense, where he studied the effects of nuclear weapons. He would go on to do research on strategy, game theory and optimisation, and played a role in starting several new companies. It’s unclear to what extent Everett’s conscious decision not to apply for professorial positions was motivated by criticism of his upstart new theory, or simply by impatience with academia in general.
He did, however, maintain an interest in quantum mechanics, even if he never published on it again. After he defended his PhD and was already working for the Pentagon, Wheeler persuaded Everett to visit Copenhagen for himself and talk to Bohr and others. The visit didn’t go well; afterward Everett judged that it had been ‘doomed from the beginning’.
Bryce DeWitt, an American physicist who had edited the journal where Everett’s thesis appeared, wrote a letter to him complaining that the real world obviously didn’t ‘branch’, since we never experience such things. Everett replied with a reference to Copernicus’s similarly daring idea that the Earth moves around the Sun, rather than vice-versa: ‘I can’t resist asking: do you feel the motion of the Earth?’ DeWitt had to admit that was a pretty good response.
After mulling over the matter for a while, by 1970 DeWitt had become an enthusiastic Everettian. He put a great deal of effort into pushing the theory, which had languished in obscurity, toward greater public recognition. His strategies included an influential article in Physics Today in 1970, followed by an essay collection in 1973 that included at last the long version of Everett’s dissertation, as well as a number of commentaries. The collection was called simply The Many-Worlds Interpretation of Quantum Mechanics, a vivid name that has stuck ever since.
Presumably nature doesn’t work like that; it’s just quantum from the start
In 1976, Wheeler retired from Princeton and took up a position at the University of Texas, where DeWitt was also on the faculty. Together they organised a workshop in 1977 on the Many-Worlds theory, and Wheeler coaxed Everett into taking time off from his defence work in order to attend. The conference was a success, and Everett made a significant impression on the assembled physicists in the audience. Wheeler went so far as to propose a new research institute in Santa Barbara where Everett could return to full-time work on quantum mechanics, but ultimately nothing came of it.
Everett died in 1982, aged 51, of a sudden heart attack. He had not lived a healthy lifestyle, over-indulging in eating, smoking and drinking. His son Mark Oliver Everett (who would go on to form the band Eels) has said that he was originally upset with his father for not taking better care of himself. He later changed his mind:
I realise that there is a certain value in my father’s way of life. He ate, smoked and drank as he pleased, and one day he just suddenly and quickly died. Given some of the other choices I’d witnessed, it turns out that enjoying yourself and then dying quickly is not such a hard way to go.
But physics hasn’t forgotten him; if anything, Everett’s ideas are more relevant than ever. His attempts to understand quantum cosmology were ahead of their time, but modern physics has made slow but steady progress on appreciating how to reconcile gravity with quantum theory. And Everett was right; once the whole Universe is your subject of study, it doesn’t make much sense to carve out a special place for a classical observer.
In my own research, I’ve gone even farther, arguing that the quest for quantum gravity is being held back by physicists’ traditional strategy of taking a classical theory (such as Albert Einstein’s general relativity) and ‘quantising’ it. Presumably nature doesn’t work like that; it’s just quantum from the start. What we should do, instead, is start from a purely quantum wave function, and ask whether we can pinpoint individual ‘worlds’ within it that look like the curved spacetime of general relativity. Preliminary results are promising, with emergent geometry being defined by the amount of quantum entanglement between different parts of the wave function. Don’t quantise gravity; find gravity within quantum mechanics.
That approach fits very naturally into the Many-Worlds perspective, while not making much sense in other approaches to quantum foundations. Niels Bohr might have won the public-relations race in the 20th century, but Hugh Everett appears ready to pull ahead in the 21st.
This is an edited extract from ‘Something Deeply Hidden: Quantum Worlds and the Emergence of Spacetime’ by Sean Carroll, published by Dutton, an imprint of Penguin Publishing Group, a division of Penguin Random House LLC, a division of Penguin Random House LLC. Copyright © 2019 by Sean Carroll.