The job of a chemist is not particularly fancy. Before I switched to the philosophy of chemistry, I spent many hours in a lab mixing solutions and waiting for reactions to happen. I saw how often chemists had to get their hands dirty (quite literally) trying out new things, combining materials with unpredictable reactions, and sometimes even risking their wellbeing. For sure, this messy work has improved our lives in many ways: from the food we eat to life-saving drugs. Yet when it comes to questions about the beginning of the Universe, the ultimate constituents of matter or the evolution of life, no one really goes to a chemist for answers. Biology can stake some claim to the big questions of existence but, really, it’s physics that underlies it all. As philosophers would put it, it is physics that discovers the fundamental laws of nature.
However, it would be wrong to dismiss chemistry as the poor cousin of the sciences. The knowledge produced in chemistry is essential to form a complete and accurate picture of nature, of how it works and of our place in it. To state it more boldly, there are chemical laws too. These laws can be found hidden within the periodic table, or in the behaviour of chemical reactions. They may not be recognised as laws in the same way as the laws of motion, thermodynamics or general relativity, but they underpin our understanding of the world. Spanning the small to the big, they can help explain everything from why a gold wedding ring does not rust to the emergence of life on Earth.
The idea of ‘laws of nature’ has a long and intriguing history. Some scholars believe that René Descartes established the existence of natural laws in their modern sense. Others track the idea to Johannes Kepler’s astronomical writings, while there is also mention by Seneca, Ovid and William Shakespeare. For example, in The Tragedy of Cymbeline, Shakespeare calls it a natural law that a fetus stays in a mother’s womb for nine months, insinuating the existence of a biological law. The difference in opinion about origins lies in their usage outside scientific enquiry. Apart from being related to the judiciary, laws have also been strongly associated with the divine ruling of (some) God, such as the Ten Commandments. These legal and theological definitions led philosophers and scientists (at least up until the 17th century, if not longer) to understand the term metaphorically as prescriptions or commandments set by nature. This was, for example, Kepler’s attitude towards his astronomical laws.
Today, philosophers agree that laws serve a special function in science. They describe phenomena or events that always happen in a certain way, under specific conditions. They recur regularly; hence they are sometimes called ‘regularities’. Of course, there are many things that happen regularly. You wake up every morning, for instance, but you wouldn’t take this to hold any special weight. However, if you throw the alarm clock across the room, it will follow a law as it falls to the ground: the law of gravity. In technical language, this is a statement about how bodies with masses attract each other, with a force that is proportional to the product of their masses and inversely proportional to the square of their distance. The broader point is that this regularity is non-accidental, and science is in the business of understanding such regularities in terms of laws. The law of gravity explains not just the fall of alarm clocks, but also the orbits of the planets, the eclipses that occurred 2,000 years ago and the tidal forces in the seas. It offers more than an explanation for all these events; it unifies them.
This is what makes laws of nature so important. By uncovering them, scientists build a systematic and predictive framework through which we can understand how seemingly disparate things in space and time behave and interact.
Physicists would say that chemistry is just applied physics; chemists would say that biology is just applied chemistry
In light of this, it is no surprise that physics is seen as uncovering laws of nature. The three laws of motion, the laws of mass conservation, Coulomb’s law and the laws of thermodynamics are some characteristic examples. But in the other sciences too, one comes across the mention of laws.
In biology, there is ‘Mendel’s law of dominance’, while in economics there is the ‘law of supply and demand’. In chemistry, you will find ‘the law of definite proportions’, coined at the turn of the 18th century, which states that a chemical compound always contains the same elements in the exact same proportions by mass. As we’ll see, I believe it’s not the only law in chemistry.
However, whether any of these statements are regarded as genuine laws, like physical ones, is controversial. This is often revealed by scientists’ attitudes towards fields of study. Personally, I came across it when I was studying chemical engineering, which brought me into contact with experts in physics, mathematics, chemistry and biology. They had divergent views on how their field is placed among the rest. The physicists were a bit snobbish towards chemists, and so were chemists towards biologists. Everyone claimed some form of superiority. Physicists would say that chemistry is just applied physics; chemists would say that biology is just applied chemistry; biologists would claim that life’s complexity cannot be captured solely by analysing atoms and molecules. A cartoon even circulated that captured that competition. Funnily enough, in the illustration, it is the mathematician who ultimately wins the game, supposedly practising the purest form of scientific enquiry.
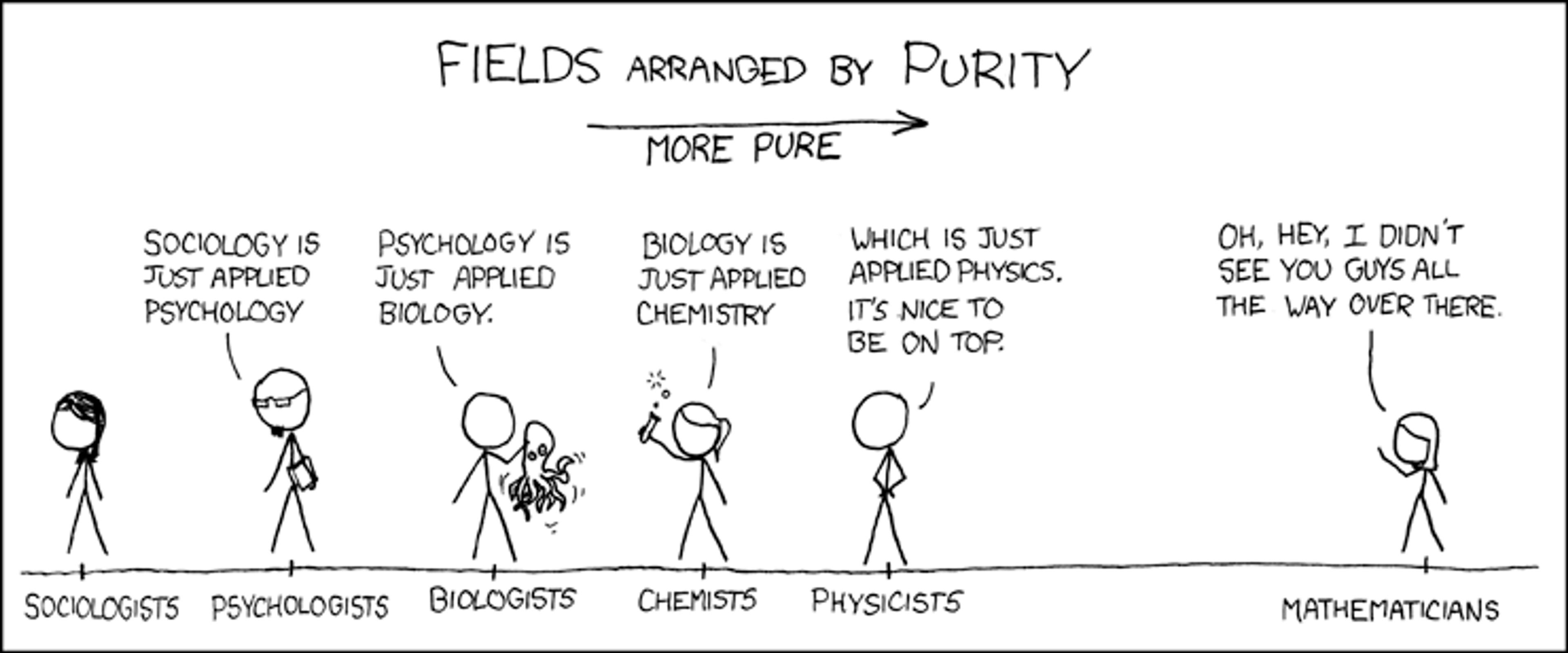
Courtesy XKCD
At the time, I didn’t think much of this. But in later years, philosophising about science made me consider this tension from an entirely new perspective. I realised that, even if physicists don’t acknowledge it, a feeling of superiority over other sciences stems from the belief that physics alone contributes to the discovery of genuine laws.
Philosophers often explain this in terms of the fundamentality of physical laws. As Walter Ott puts it in The Metaphysics of Laws of Nature (2022), ‘laws have to be “fundamental” to the science; they typically shouldn’t appear as downstream corollaries to other propositions’. This undermines the idea that there are any genuine laws outside physics. In the case of chemistry, this is due to quantum mechanics: you could describe all elements and compounds in terms of the behaviour and interaction of their constituent particles. There is no need to invoke further laws from chemistry. In his essay ‘Why There Are No Laws in the Special Sciences’ (2010), Jaegwon Kim says it this way:
If the phenomena investigated by the special sciences are part of the all‐encompassing physical domain, how can there be special‐science laws and explanations in addition to physical laws and explanations? That is, how are special sciences possible? And even if they are possible, do we need them? Why shouldn’t developed physics meet all our needs?
Reading this, it’s easy to understand the snobbishness of physicists. If physics uncovers the fundamental laws of the Universe, then it seems to follow that all other statements we formulate in chemistry – or indeed any other science – must stem from those.
However, this overlooks that nature operates at different scales. Once we look at systems larger than single atoms, their complexity makes it hard to describe them in terms of electrons, protons and neutrons. What’s more, they exhibit patterns that can be revealed only through a different lens, including the chemical one. This is most characteristically shown by two – surprisingly neglected – cases in chemistry: the periodic table and chemical reactions.
First, consider the periodic table. It is no exaggeration that the periodic table is one of the most popular representations in science. Who hasn’t come across a poster of the table, or heard catchy songs that help memorise its elements? The table is a picture of all known chemical elements (118 so far) and while it may seem to be set in stone nowadays, this was not always the case. Back in the 19th century, chemists debated about which classification was best, entering into disputes about priority and recognition. Dmitri Mendeleev, John Newlands and Lothar Meyer were the main protagonists, with Mendeleev’s proposed table eventually winning over the rest. Each contender claimed to fulfil a specific virtue with their proposed classification. Newlands’s table achieved simplicity: the relation underlying the elements’ ordering was a simple mathematical one between elements’ atomic weights. Meyer’s table paid close attention to the quality of the available empirical data, excluding elements for which the data was still considered unreliable. Mendeleev achieved completeness. That is, his table included almost all known elements of his time. It was accepted over the others because his proposed classification did something the others couldn’t: predict the existence of elements that scientists had not discovered yet.
In Mendeleev’s original version, the elements were positioned in terms of atomic mass. Today, it’s atomic number, since it was found that elements are more accurately identified through the number of protons in their nucleus. Starting with hydrogen at the top left – with one proton in its nucleus – elements are placed consecutively from left to right and top to bottom. But this is not all. It just so happens that, by placing elements in that order, they exhibit certain patterns in their properties. Elements found in the same column and the same row have certain physical and chemical properties in common. For example, materials that are made of alkali metals (ie, of any element of the first column, excluding hydrogen) are soft and malleable. Elements of the fourth row of the table are quite stable: they don’t react very easily. Such information is particularly handy because it helps chemists explain phenomena and make predictions about how materials interact with one another.
The periodic table’s value did not escape early chemists. From the very beginning, they believed that what they had achieved was not just the formulation of a useful classification but the discovery of a law, the so-called ‘periodic law’. In his paper ‘Periodic Law’ (1871), Mendeleev talked about ‘the law of periodicity … that was applicable to study the relations between the properties and the atomic weights of all of the elements’. Newlands too, in his attempt to claim priority, said that he was ‘the first to describe the periodic law, showing the existence of a simple relation between them when so arranged’. Nowadays, the periodic law refers to the fact that, when elements are positioned in terms of increasing atomic number, there is an approximate recurrence of their physical and chemical properties.
‘Gold is unreactive’ is a universal statement that doesn’t concern a particular chunk of gold
But it is not so much the periodic law that makes the table so significant. Through the periodic table, chemists uncover regularities about how different chemical elements (and sets of them) behave and interact with each other. Statements such as ‘alkali metals are soft and malleable’, ‘gold is shiny’ or ‘halogens are very reactive’ are all statements of non-accidental regularities that are represented and derivable by analysing the periodic table. As such, I believe they should be granted lawful status in the same way as physical laws are.
To convince you of this, we need to see how philosophers unpack the idea of laws in science. All more or less agree that there are certain features exhibited by all statements of laws in science. For example, a statement of a law (such as the law of gravity) does not refer to a specific event in space and time, nor to a specific object or particle with mass, but to all objects that have certain properties (in this case, mass). They are also universal (or at least statistical generalisations), meaning that they hold for any object of the relevant properties, anywhere and at any time. In addition, laws are used to make predictions. For example, by invoking the law of gravity I can predict that my thrown alarm clock will fall to the ground. It will also help me explain why. Lastly, laws allow us to make counterfactual claims of the form ‘If – then’. For example, the law of gravity allows me to examine whether hypothetical situations are possible. For instance, if I threw an elephant from the balcony, would it fall?
These criteria are satisfied by the statements that chemists make about the elements in the periodic table. Take for instance ‘gold is unreactive’. This is a universal statement that doesn’t concern a particular chunk of gold exclusively but allows us to make inferences about any chunk found at any time and place. In fact, it explains why all the golden necklaces made in ancient Egypt have not spoiled after thousands of years, and it allows us to predict that any gold that astronauts might discover on a distant Earth-like planet will also be shiny and stable. Lastly, it allows us to make counterfactual claims of the form ‘If my necklace were made of gold, then it would not have rusted.’
But it is not just the periodic table that uncovers laws of nature. After all, if statements of the form ‘halogens are very reactive’ represent laws, then so do statements of the form ‘acids react with bases to form salt and water’. That is, we should admit all statements about chemical reactions as lawful, whether they concern transformations between atoms or between molecules. Chemical reactions play a huge part in chemistry: their understanding defines the discipline. From how our gut processes food to how flowers absorb sunlight, our way of life depends on chemical reactions happening both spontaneously and through human intervention. The ability to manipulate these transformations hinges on having a robust understanding of the relevant chemical laws. After all, the enormous success of chemistry – both theoretical and practical – would be miraculous if statements on chemical reactions referred to merely accidental regularities.
Does all this suffice to convince us that chemical laws hold the same status as physical ones? Consider again ‘gold is unreactive’. In this case, we can ask whether Schrödinger’s quantum equation captures all we want to say by the statement that gold is unreactive. I do not believe it does. Let me put it this way. Imagine there was no chemistry and that we described chemical phenomena exclusively in terms of how protons, neutrons and electrons interact. Would we miss out part of the picture of how things work? Even if everything is the result of the interactions between subatomic particles (which I do not contest), I think we would.
Chemical laws are necessary to fully appreciate the richness inherent in the transformations of inanimate matter, as well as the emergence of life itself. As such, they are no less significant than the law of gravity, the law of relativity or the laws of thermodynamics. They hold the same predictive and explanatory power as paradigmatic laws of physics and should be recognised as part of the story we tell when addressing the big questions about life and the Universe.