Space, as they say, is big. In The Hitchhiker’s Guide to the Galaxy (1979), Douglas Adams elaborates: ‘You may think it’s a long way down the road to the chemist, but that’s just peanuts to space.’ It’s hard to convey in everyday terms the enormity of the cosmos when most of us have trouble even visualising the size of the Earth, much less the galaxy, or the vast expanses of intergalactic space. We often talk in terms of light-years – the distance light can travel in a year – as though the speed of light is somehow more intuitive than a number written in the trillions of kilometres. We give benchmarks in the same terms (it takes light 1.3 seconds to travel between the Earth and the Moon) but, in our everyday experience, light is instantaneous. We might as well talk about the height of a building in terms of stacking up atoms.
Maybe, if we’re feeling more adventurous, we use analogies based on personal experience. The distance to the Moon is 32 million school buses! If you could drive there in one of those school buses, going at 60 miles per hour, it would take you 166 days! I’m not sure that helps.
I wish I could say that astronomers have a better intuitive grasp of all this. We don’t. Brains don’t really work that way. So we cheat with numbers. We use longer yardsticks to talk about bigger spaces: kilometres, light-years, parsecs, kiloparsecs, megaparsecs, gigaparsecs. We get comfortable with exponents (1,000 is 103; 1 trillion is 1012) and think in logarithmic intervals, where each successive step is a new power of 10. At some point, distance stops being a straightforward concept entirely. Here in the Solar System, space and time are both more or less well-behaved, but when you have to deal with the cosmos as a whole, you have to factor in the fact that it refuses to sit still for its fitting.
Space is expanding. It has been since the Big Bang, and it’s not stopping any time soon. If you look at a galaxy far, far away, not only do you have to factor in that the image you’re looking at is old, you have to account for the fact that it’s no longer where it was when you saw it. Let’s say you see a supernova go off, in a galaxy a billion light-years away. Did the supernova just go off, or did it go off a billion years ago? You can say the latter, because the light has been travelling to us for a billion years, but since there was no way to observe it back then, what does saying that it went off in the past even mean? And that billion-light-year-distant galaxy – how far away is it, really? Maybe a billion years ago it was a billion light-years away, but the Universe has been expanding all that time, so now it must be much farther. Which distance do we use?
Even time is distorted by the stretching of space. We can watch the brightening and dimming of that exploding star, as the shockwave tears through it, and say it took about 100 days to fade away. But if we compare it with a supernova nearby, on average, we’ll see that the distant one takes a few days longer. From our perspective, it’s exploding in slow motion.
Even with the limitations of definition, we do our best to measure our space and quantify its farthest reaches. We have catalogued countless galaxies, some so distant that their light has taken almost the entire lifetime of the cosmos to reach us. We have searched our maps of the cosmos for some indication of an edge, or a centre, and found none. We have no reason to believe the cosmos doesn’t just go on forever, in every direction, without any significant change in content or structure. Our galaxy is a single grain of sand in a vast unbroken desert; zoom out far enough, and everything looks more or less the same.
There is a limit, though. However powerful our telescopes, and however long we stare, we will never see anything farther away than the edge of the cosmic bubble we call our ‘observable universe’. This is an imaginary sphere, centred on us, and defined by the speed of light and the age of the cosmos. The radius of this bubble is the distance that a beam of light could cover if it travelled for the entire age of the Universe.
If every time we look out into the cosmos, we’re looking into the past, it stands to reason that looking far enough away could mean looking at the time so far into the past, it’s the moment when the Universe first formed. That’s what defines our cosmic horizon. Put another way, anything beyond our cosmic horizon is so far away that even if a light beam left it at the very moment the Universe started, 13.8 billion years ago, the distance is so vast that the light beam hasn’t had time to reach us yet. There hasn’t been enough time.
When we look at the edge of the observable universe, we see a cosmos that is still on fire
We have good reason to believe that in this apparently boundless universe, there are galaxies beyond the horizon, just as, when you stand on the ocean shore and see nothing but water, you have reason to believe there’s land out there eventually, beyond what you can see. If you jumped in a ship and sailed away, your horizon would move with you, and you would eventually see that land. Similarly, if you could take off in an interstellar rocket ship to another part of the cosmos, your horizon would still be centred on you, wherever you were. Unfortunately, limited as we are by the laws of physics and the constraints of our modes of travel, getting far enough from home to significantly change our field of view isn’t practical. But we can still make inferences about what might lie beyond it. And despite the cosmic horizon being as subjective a boundary as a horizon is on Earth, it has one very important difference.
When we look out to the edge of the observable universe, what we see is something truly astounding. The most distant light is also the oldest; it’s the light from the Big Bang itself. The early universe, right after the first moments of creation, was hot and dense, everywhere, humming with vibrating plasma; right at the edge of our vision, we’re looking into the past so far that we literally see that glowing plasma. The inferno persisted for around 380,000 years before space expanded and cooled enough that light and particles could travel freely through it. When we look at the edge of the observable universe, we see the last smouldering embers of that hot dense phase. We see a cosmos that is still on fire.
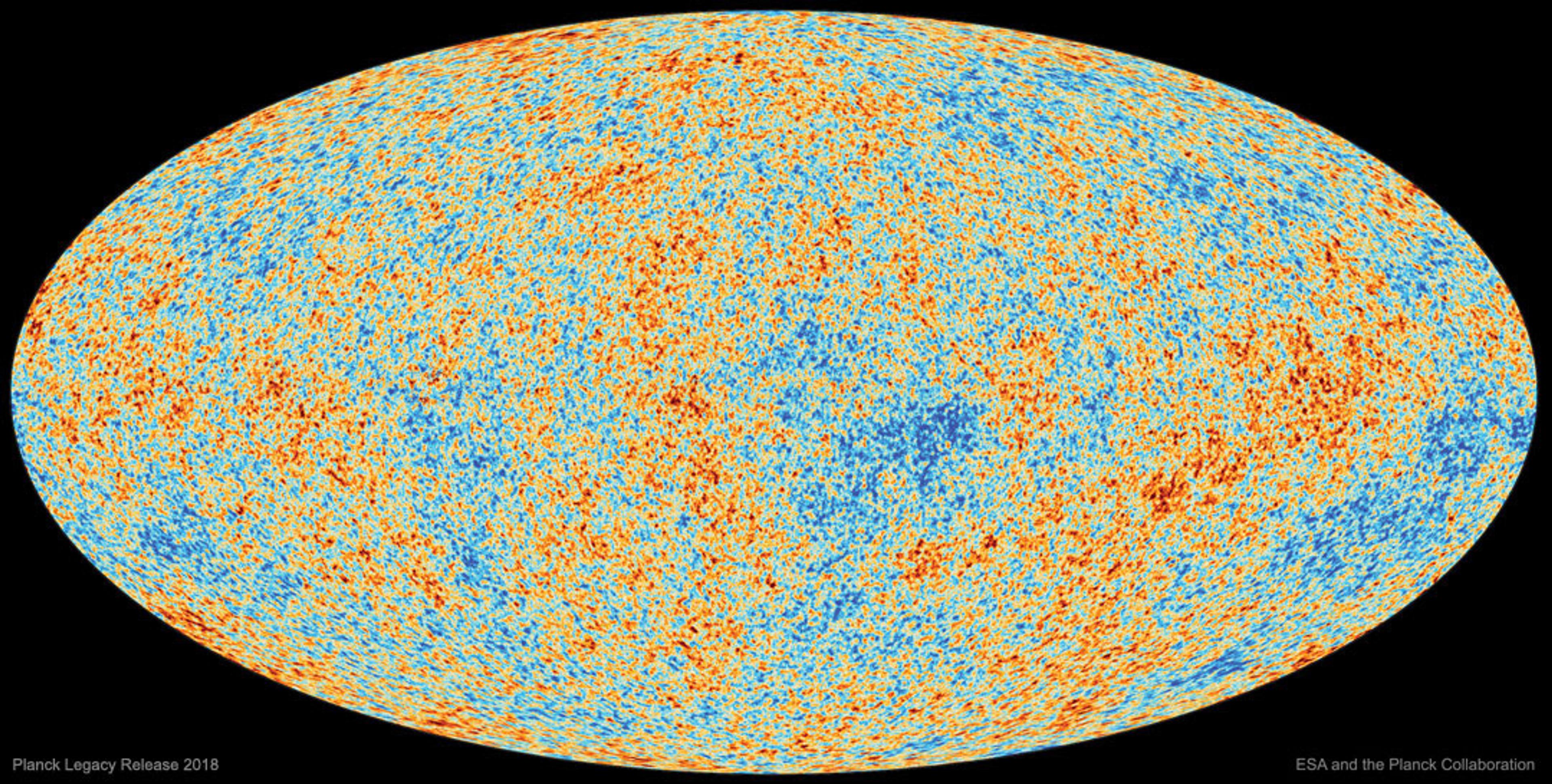
The cosmic microwave background (or CMB) as seen by the ESA’s Planck space-based observatory. The CMB fills the entire Universe and is leftover radiation from the Big Bang. Courtesy ESA/Planck.
The distance to our cosmic horizon is not, as you might expect, 13.8 billion light-years. As we discussed above, distances are weird in an expanding universe. Something that was 13.8 billion light-years away when its light started the journey toward us is much farther away now. If you factor all that in, that glowing plasma we see at the very edge of the observable universe is actually somewhere around 45 billion light-years away now.
Just because we can’t see things beyond our fiery horizon, it doesn’t mean that there’s nothing there. The evidence we have, studying the same-ness of galaxies in every part of the cosmos we’ve mapped, points to the notion that space continues far beyond our horizon, in every direction; the limits to our vision are circumstantial. If we happened to live in a galaxy that lies just outside our current horizon, everything we know of the cosmos suggests that our view from there would look pretty similar to the view we have from here. Very distant reaches of the cosmos could, in principle, be totally different, of course – we can’t know for sure without being able to see them. In fact, regions far enough beyond our horizon can even be considered to be separate, isolated universes of their own, for all practical purposes, since they can’t interact with ours.
But what if the universe isn’t just bigger than we perceive, circumstantially, but bigger than we even can perceive? What if it extends in every direction, and then some?
Our everyday experience tells us that space has three dimensions. Front/back, left/right, up/down. In physics, we describe time as a fourth dimension, and wrap the whole thing up as spacetime – a kind of bendy 4D cosmic grid. The malleability of spacetime, a basic tenet of Albert Einstein’s relativity, allows that grid to warp and stretch in response to the motion and mass of everything in it. It’s why space can expand and distort the passage of time, and why time moves more slowly if you’re in a fast rocket or hanging out near a black hole.
However, physicists have been wondering for years whether the three dimensions of space we experience might be only part of the picture. If there are more spatial dimensions, extending out in new directions we can’t perceive, that could help to explain some puzzling aspects of theoretical physics and the behaviour of gravity. Add an extra dimension of space to the Universe, and you find that gravity can ‘leak’ out into it, making the force seem weaker than it really is, and potentially explaining why its strength is so minuscule compared with the forces governing particle physics.
What if an extra dimension can hide an entire universe?
Higher dimensions of space are also a requirement for string theory, which postulates that what we see as elementary particles are actually strings of energy vibrating in several more dimensions than we can see. In those theories, the extra dimensions are ‘compactified’ – curled up on themselves – so that if you did manage to find one of those new directions and set out on a journey, you wouldn’t be able to go very far before you ended up right back where you started.
But what if an extra dimension can hide an entire universe?
One hypothesis for the structure of our cosmos, developed in the early 2000s, suggests that we might live in a three-dimensional ‘brane’ (think: membrane) on the edge of a larger space with four spatial dimensions (plus time). Inside that higher-dimensional ‘bulk’ there could be another 3D brane, containing another universe, that might, from time to time, come crashing into our own. This theory’s originators called it the ‘ekpyrotic’ model of the cosmos, after a Greek term for conflagration – a nod to the fact that each cosmic collision would result in the fiery conditions of the Big Bang, and could explain the origin and eventual fate of our universe. In this model, the branes alternately move toward each other, collide and then move apart again, in an endless cycle, going from Big Bang, to expansion, to Big Crunch, and back to Big Bang. The patterns of structure we see in the cosmos today (distributions of galaxies and clusters) are, in this model, seeded by the interaction between the two branes in the slow collapse phase before the Bang.
While it might seem extravagant at first glance to postulate higher dimensions and new universes just to explain the Big Bang, there are good reasons why physicists take these ideas seriously. The standard picture of the early universe is probably more complicated than you’ve been led to believe. When you think of the Big Bang, the first thing to come to mind is likely to be a singularity – an infinitesimal point of infinite density containing all of space and time that suddenly explodes out to create the entire cosmos. That idea became popular because Einstein’s equations of gravity can describe a cosmos that begins that way (and perhaps wraps itself up with a Big Crunch singularity at the end), but it doesn’t work with what we see in our observations. That background light we see right at the cosmic horizon, that afterglow of the Big Bang, tells us that a simple evolution from a singularity to the big beautiful universe we enjoy now just doesn’t make sense.
The problem is that the Big Bang’s afterglow, what we refer to as the cosmic microwave background, is too perfect. To an absurd degree of precision (one part in 100,000), it looks the same, in every direction. Same colour (or, rather, frequency, since it’s microwave light), same spectrum, same intensity. The reason that’s a problem is because there’s no reason why two regions on opposite sides of the sky should match in that way. Even if everything started together, wrapped up in a singularity, the way it expanded outward should have introduced extreme differences in different parts of the early cosmos. Regions that are now far apart from each other in the expanding fireball stage of cosmic evolution never had a chance to come to an agreement on what temperature to be. The cosmic microwave background should be drastically different on one side of the sky than it is on the other.
The explanation that physicists settled on in the 1980s inserted a new chapter into our cosmic story. What if, they said, in the very early cosmos, before the hot fireball stage, there was a period of extremely rapid expansion? Maybe right after the singularity (assuming there was one), the early cosmos really was a patchy mess – much hotter in some places, and cooler in others. But then the cosmos expanded so rapidly that one tiny patch, too small to have much variation, stretched out until it was large enough to make up our entire observable universe. Then, the physicists hypothesised, whatever weird new component of the Universe caused that super-stretching (we call it ‘cosmic inflation’) suddenly decayed everywhere into radiation and ignited the expanding-fireball-cosmos visible in the background light we detect today.
From what we’ve seen so far, inflation seems to work well with our current paradigm, and even nicely explains the little one-part-in-100,000 fluctuations observed in the cosmic microwave background light. But we don’t have what anyone would call solid proof that it happened, nor can we say how or why it started, or what drove it.
And, by the way, even in the paradigm of inflation, the possibility of getting smacked by another universe is not entirely out of the question.
Two bubble universes might at some point collide, leaving imprints like bruises on each other’s background light
If inflation did happen, the sequence of events that created our observable universe could have occurred time and time again in different parts of a much larger space, in a process called ‘eternal inflation’. The idea is that the larger background space is always inflating but, once in a while, in a small part of it, inflation stops; that bit of universe heats up, and normal cosmic expansion takes over. This would create a kind of multiverse, as little bubble universes, defined by those separate post-inflation regions, continually drop out of the inflating background. Each bubble universe would be separated from the others by that constantly expanding space, and would be incapable of interacting with each other. For the most part.
Once in a while, two bubble universes might appear close together. If they do, and if each continues to expand, they might at some point collide, leaving bubble-shaped imprints like bruises on each other’s background light.
Astronomers have looked for those bruises. None have appeared so far, but we’ll keep searching. And meanwhile, some of us will continue to look askance at the inflation picture, with its unknown engine and its infinite multiverses, and try to find some more palatable alternative.
As for the ekpyrotic model, it’s undergone a number of revisions over the years, and the current version doesn’t involve higher dimensions or cosmic collisions at all. In some ways, it looks more like inflation: driven not by the motion of branes but by the evolution of a scalar field, a species of space-filling energy field similar to what most physicists think fuelled cosmic inflation. (And some new models of inflation involve brane worlds too, just to keep things interesting.) Despite no longer requiring grand cosmic collisions, the ekpyrotic model still includes a transition between a collapsing universe and a Big Bang. In the new version, though, the collapse might be a relatively modest one, resulting in a little bit of compression before the sparking of the conflagration that starts the new cycle. If it cycles on and on forever that way, rather than endless pocket universes, our larger space would be one giant, ever-growing cosmos: expanding, taking a breath, and expanding again, over and over.
The cosmic horizon defining our observable universe is a hard limit. We can’t see beyond it, and unless our understanding of the structure of reality changes drastically, we can be confident we never will. The expansion of the cosmos is speeding up; anything beyond our horizon now will be carried away from us faster and faster, and its light will never be able to catch up. While we might never be able to say with certainty what lies beyond that border, what all the theories have in common is that our observable universe is part of a much, much larger space.
Whether that space contains a multiverse of bubbles, each with different physical laws; whether it’s part of an ever-growing cosmos of which we are only one part, in one cycle; or whether space extends outward in directions we can’t conceive, we currently just don’t know. But we’re seeking clues.
The patterns in the cosmic microwave background light, the distribution of galaxies, and even experiments testing gravity and the behaviour of particle physics are giving us insight into the fundamental structure of the Universe, and into its evolution in its earliest moments. We are getting closer and closer to being able to tell our whole cosmic story. We can already see, directly, the fire in which our universe was forged, the moments just after its beginning. With the clues we are gathering now, we might, someday, follow the story all the way to its end.
This Essay is based on Katie Mack’s forthcoming book, The End of Everything (Astrophysically Speaking), publishing on 4 August 2020 by Scribner.
This Essay was made possible through the support of a grant to Aeon from the John Templeton Foundation. The opinions expressed in this publication are those of the author and do not necessarily reflect the views of the Foundation. Funders to Aeon Magazine are not involved in editorial decision-making.