I want to start with a proposition: if Earth had never come alive, it would be a profoundly different world. Conversely: the planet of today has, to a remarkable extent, been made what it is by the activities of lifeforms. Over the course of the planet’s long history, a history that extends back more than 4.5 billion years, lifeforms have shaped the rocks, the water, the air, even the colour of the sky. A Never-Life Earth would not even have as many different kinds of minerals.
This is the portrait painted by the modern science of life and Earth, a fusion of biology and geology that seeks to understand all the planets that Earth has been, and that unites such apparently unrelated fields as the study of bacterial metabolism with the physics of atmospheres. The central observation of this undertaking is that, over time, lifeforms have profoundly altered the fabric of this planet, and this, in turn, has altered the circumstances in which lifeforms evolve.
Yet the idea that lifeforms might alter Earth is not new.
In his encyclopaedic Histoire naturelle, générale et particulière (1749), the French natural philosopher Georges-Louis Leclerc, Comte de Buffon remarked on the prodigious heaps of fossil shells that make up many rock formations, and stated that substances such as limestone, chalk and marble, despite their inanimate nature, are the result of the activities of lifeforms. In Hydrogéologie (1802), Buffon’s protégé Jean-Baptiste, Chevalier de Lamarck took the point further, suggesting that the activities of lifeforms had shaped the mineral composition of Earth. But as far as I have been able to discover, the person who first attempted to measure the changes that lifeforms have wrought, and who had the clearest sense of why they have an impact, was none other than Charles Darwin. In thinking about the Anthropocene – the age of human impacts upon Earth – the lessons of his work have never been more important.
Darwin, of course, is famous for his work on evolution. His book On the Origin of Species (1859) laid out a wealth of evidence that evolution occurs, and proposed a mechanism – natural selection – for how it does so. Although much has been learned since, and many of his ideas have been extended, corrected or refined, the Origin remains the founding text of modern biology, and is the pinnacle of Darwin’s work. But Darwin’s first scientific monograph and his last – the two bookends of his thoughts, so to speak – were both about how animals have, over vast spans of time, transformed the landscape.
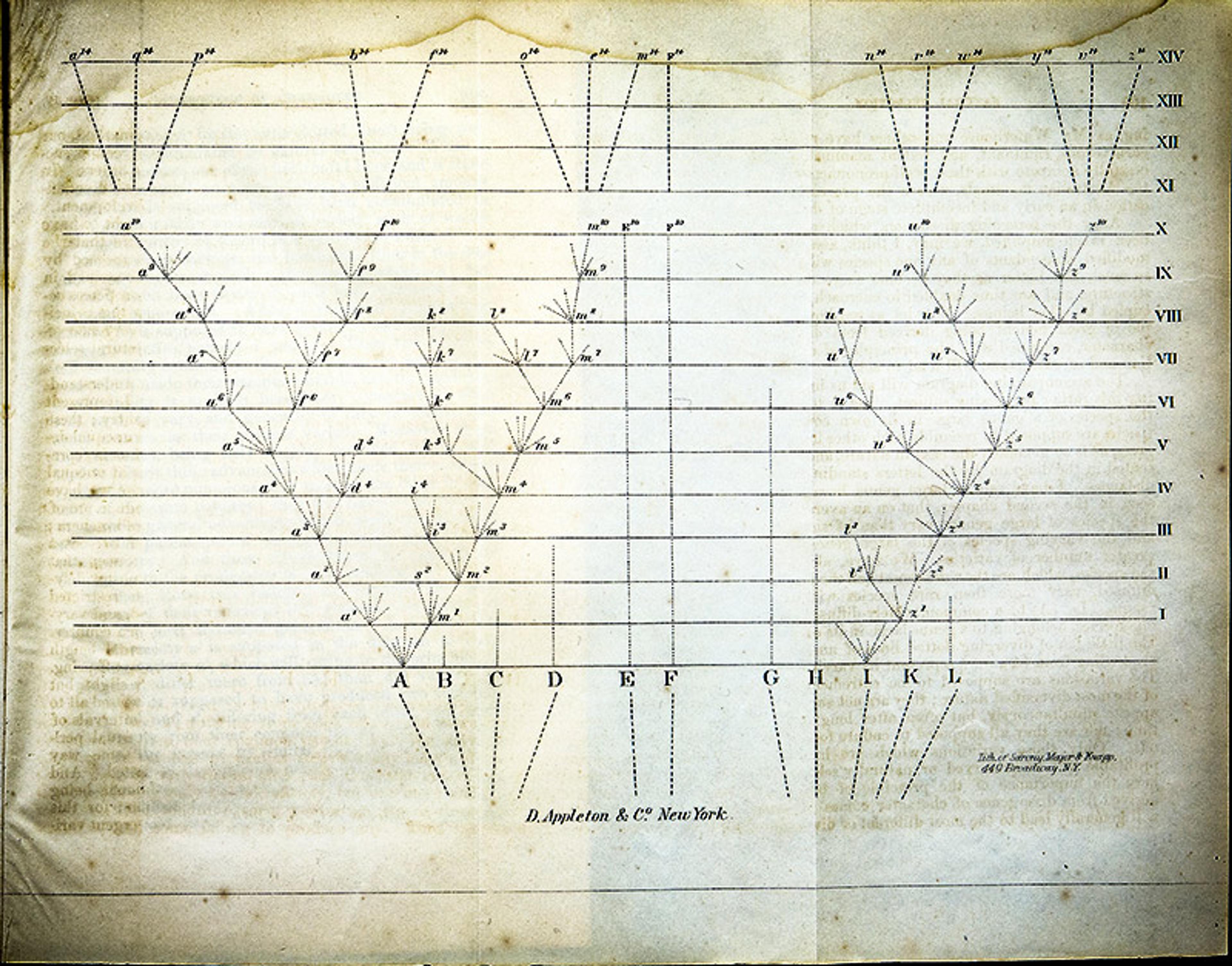
These two works of biogeology – one on coral reefs, the other on earthworms – were, as far as I know, the first detailed studies of the subject ever published. On casual inspection, they appear to be unrelated undertakings, just part of Darwin’s long and eclectic list of interests, along with barnacles, orchids, carnivorous plants, peacocks’ tails, the emotions of humans and other animals, the volcanoes of South America, and so on. This impression is enhanced by the fact that the two works differ greatly in style and were published almost 40 years apart – The Structure and Distribution of Coral Reefs appeared in 1842, while his book on earthworms, The Formation of Vegetable Mould, Through the Action of Worms, with Observations on Their Habits, came out in 1881, about six months before he died. (Vegetable mould is what you and I would call topsoil.) But, actually, these two books reflect life-long interests and, taken together with his work on evolution, show a vision of Earth astounding in its completeness and magnificent in its scope. Darwin had an extraordinarily complete conception of the workings of the world, anticipating current ideas of the co-evolution of life and Earth by more than a century.
All his life, Darwin was interested in the power of huge numbers of small things – acting slowly, over years, decades, and centuries piled upon centuries – to have enormous impacts. In this, he was inspired by the work of Sir Charles Lyell, who had argued that geological change takes place through slow, gradual processes accumulated over vast spans of time. Darwin took that idea and applied it to biology, making it the unifying insight of his work on corals, evolution and worms. It’s a simple insight, yet one that is strangely hard to develop a feeling for. The human brain has evolved to think in terms of hours, days, months, perhaps a few years. Conceiving of changes summed over decades, centuries, millennia and on into the dizzying spans of Earth history – that’s far more difficult and elusive. A single earthworm has no particular significance. But given enough worms and time, Darwin argued, entire landscapes would be transformed. Coral animals are likewise minute; nonetheless, with an abundance of them, plus a few million years, corals can build gigantic structures. As Darwin remarked:
We feel surprise when travellers tell us of the vast dimensions of the Pyramids and other great ruins, but how utterly insignificant are the greatest of these, when compared to these mountains of stone accumulated by the agency of various minute and tender animals!
He wrote these words in The Voyage of the Beagle (1839), an account of his adventures during a five-year circumnavigation of the globe. The ship sailed from England on 27 December 1831, when Darwin was just 22. In the course of this voyage, he visited South America, New Zealand and Australia, as well as a number of small islands, including Cape Verde, the Galápagos, Tahiti, Cocos (Keeling) and Mauritius.
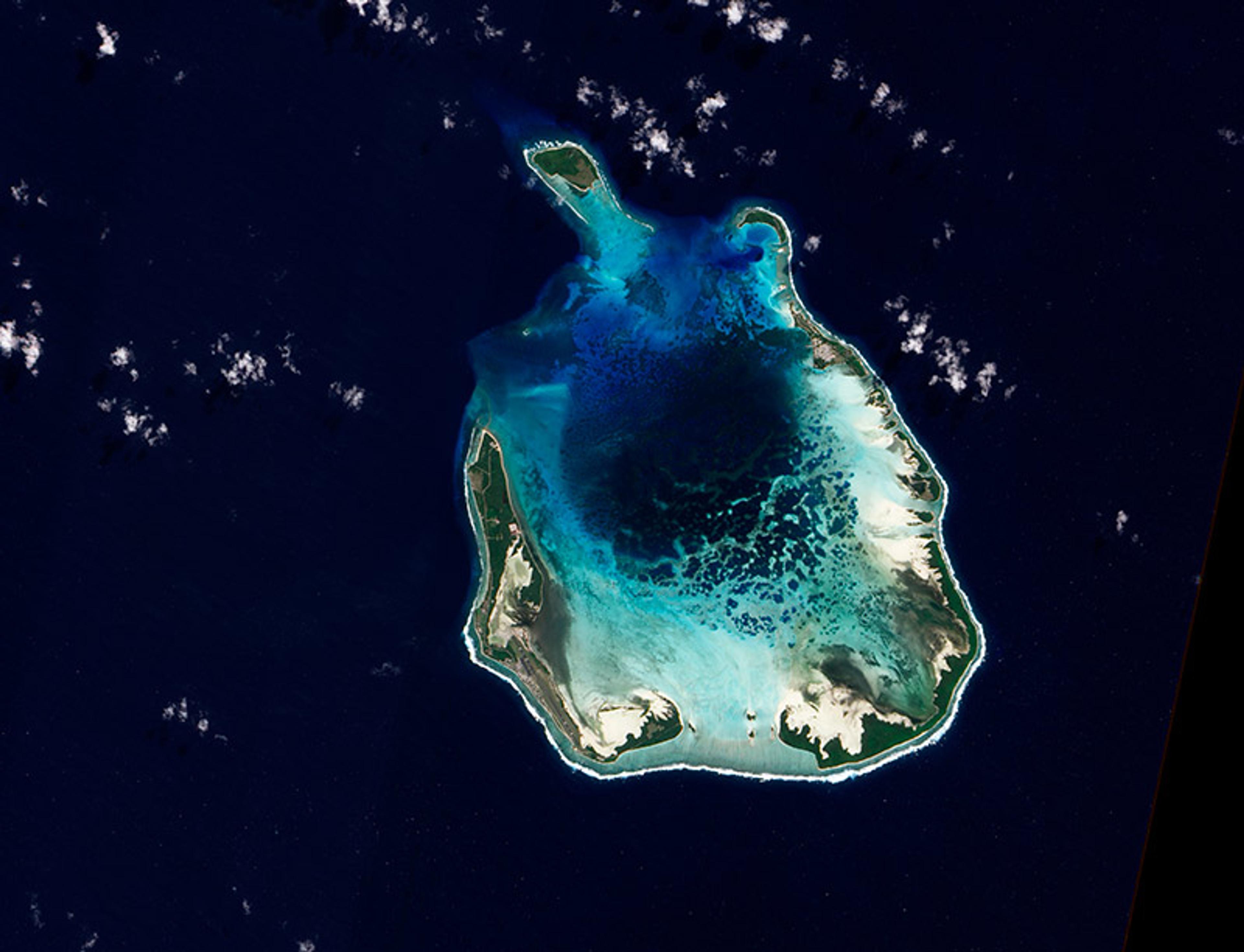
During the voyage, Darwin developed a theory of the formation of coral atolls – those rings of low, flat islands around a central lagoon – that dot the Pacific and Indian oceans. Remarkably, he developed his ideas before he had ever visited an atoll, or seen a reef, working instead by a process of deduction from observations he made while studying the geology of the west coast of South America. An opportunity to inspect an atoll in person didn’t come until many months later, when the HMS Beagle called at the islands of Cocos (Keeling), in the Indian Ocean, in April 1836, just six months before the ship would arrive back in England.
Each atoll is a requiem for a volcano. Or, as Darwin wrote – ‘a monument over an island now lost’
The ship spent 12 days at the islands, giving Darwin plenty of time to explore. One day, when the sea was calm and the tide was low, he propelled himself to the outer edges of the reef ‘by the aid of a leaping-pole’, curious to examine the corals that ‘break the violence of the open sea’. I like to think of him there, youthful and vigorous, splashing in the surf against a backdrop of coconut palms and an azure sky, colourful fish darting in the calm waters of the lagoon nearby.
Atolls have a number of puzzling features. First, they are all low and flat. As Darwin remarked, ‘there are enormous areas in the Pacific and Indian Oceans, in which every single island is of coral formation, and is raised only to that height to which the waves can throw up fragments, and the winds pile up sand.’ Second, they appear suddenly in the deep parts of the ocean. Third, they tend to cluster. And finally, since they are built by lifeforms that like to live in warm, sunny, shallow waters, and yet occur where the ocean is deep, each must be constructed on top of some kind of submarine structure.
At the time, those structures were imagined to be the rims of craters of submerged volcanoes, an idea Darwin found ‘monstrous’, and ‘almost too preposterous to be mentioned’. He scorned it, in part, because individual atolls can measure more than 130 kilometres (80 miles) across – which would assume the presence of hundreds of volcanic craters of unheard-of dimensions all close to, but miraculously never jutting above, the surface of the sea.
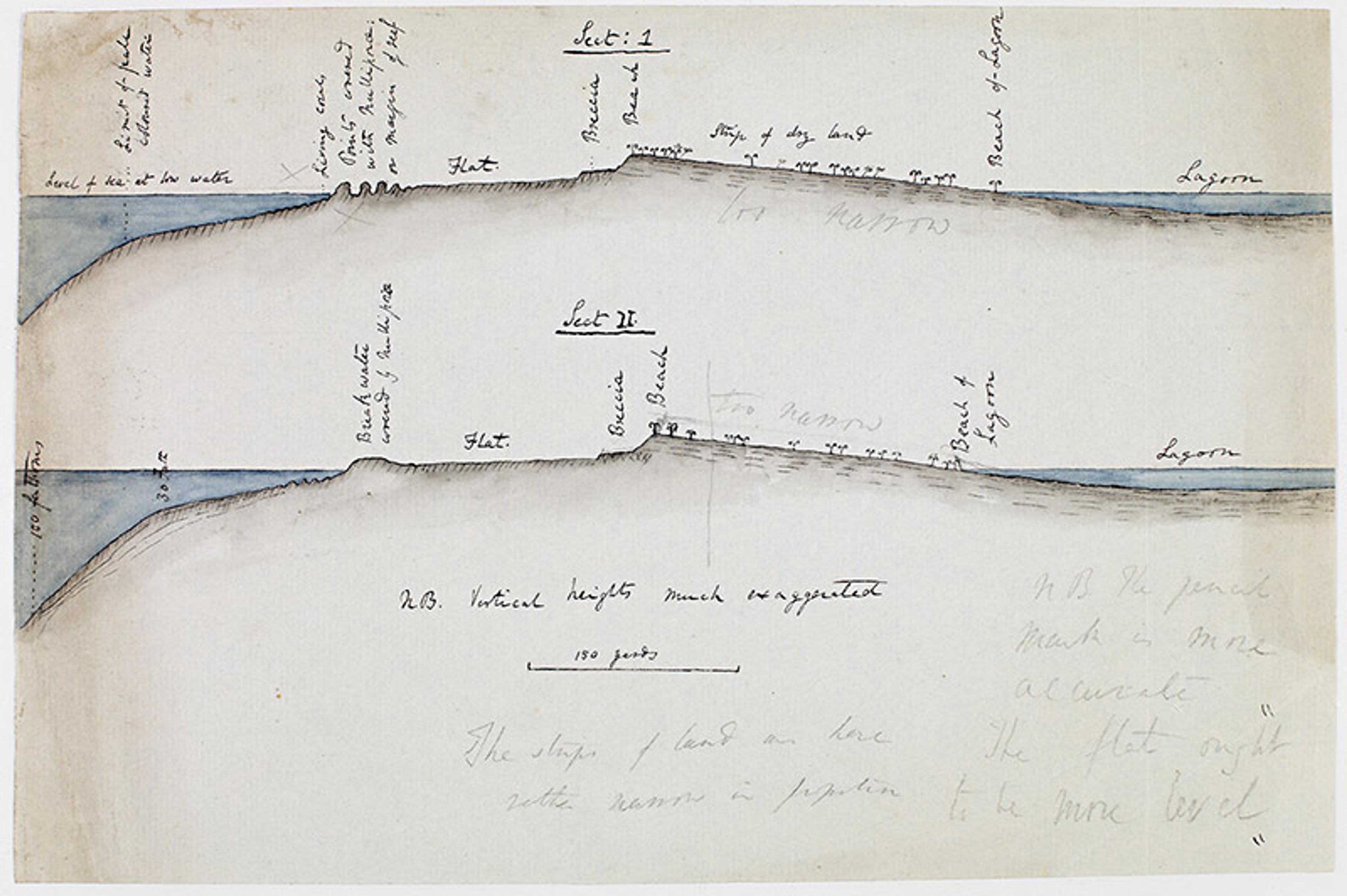
Darwin suggested instead that atolls form on the slopes of volcanoes that are slowly sinking. Here’s a brief sketch of the idea. A volcano erupts and builds an island in the middle of the ocean. Coral animals settle on its slopes, luxuriating in the warm, shallow, sunlit waters, and begin building the limestone skeletons that, together, will form a reef. If the seafloor then begins to subside, the volcano will gradually sink back beneath the waves – but the corals will continue to grow upwards, so as to remain in the shallows. As long as the volcano doesn’t sink too fast, the corals can keep pace with its descent. On the west coast of South America, Darwin had observed earthquakes heaving chunks of land upwards; here, he inverted the idea, and considered what would happen if the land should sink.
The fact that each island is built by lifeforms has an important corollary. Stone is hard; but given enough time and enough feet, stone steps can be worn to nothing. Yet in the case of atolls, the stone is continually rebuilt. Or, as Darwin put it:
The ocean throwing its waters over the broad reef appears an invincible, all-powerful enemy; yet we see it resisted, and even conquered, by means which at first seem most weak and inefficient … It is impossible to behold these waves without feeling a conviction that an island, though built of the hardest rock, let it be porphyry, granite, or quartz, would ultimately yield and be demolished by such an irresistible power. Yet these low, insignificant coral-islets stand and are victorious: for here another power, as an antagonist, takes part in the contest. The organic forces separate the atoms of carbonate of lime, one by one, from the foaming breakers, and unite them into a symmetrical structure. Let the hurricane tear up its thousand huge fragments; yet what will that tell against the accumulated labour of myriads of architects at work night and day, month after month?
Since corals cannot survive much exposure to air, they don’t grow above the surface of the sea – which explains why atolls are so flat. Meanwhile, the waves throw up fragments of broken coral and the winds pile up sands, creating low islets and sandbars. Coconuts and other seeds get washed up and sprout, creating vegetation and, with it, the quintessential desert island. Eventually, the volcano may vanish far beneath the waves, leaving just the coral as a marker of its existence. Each atoll is a requiem for a volcano. Or, as Darwin wrote – ‘a monument over an island now lost’.
His argument makes a clear prediction: somewhere beneath each atoll, beneath these immense piles of life-built rock, these mountains of limestone, you will find the remnants of a volcano.
Darwin did not have the means to drill down through an atoll and test his idea directly. Instead, on arriving back in England, he embarked on a massive study, a kind of comparative reefology. It was an exhaustive analysis of the sort that he would deploy in his work again and again. He spent many months reading accounts of earlier voyages and poring over nautical charts, classifying coral reefs by whether they were atolls, barrier reefs or fringing reefs growing close to shore. He viewed the three types of reef as parts of a continuum, arguing that, in areas of sea-floor subsidence, reefs would begin as fringing reefs, develop into barrier reefs, and finish as atolls. In the course of his work, he compiled the first global map of coral reefs ever to have been produced.

In this computer age, where you can sit at your desk and fly over any atoll you please, even take a virtual stroll along the beach of some distant islet, Darwin’s map holds a kind of poignancy. The place names are antiquated – Australia is marked as ‘New Holland’; Hawaii, as the ‘Sandwich Isles’; the Indian subcontinent, as ‘Hindoostan’ – which serves as a reminder of how long ago he was working. To put this further into perspective, no one knew then that corals live in shallow water because they harbour symbiotic single-celled lifeforms that require sunlight to grow; the establishment of the great age of Earth was still more than a century in the future. The appendix of his book details where he got his information, and how he decided on the nature of each reef. The tone is dry and dusty, and would cure most cases of insomnia – ‘Starbuck Isld (5˚S., 156˚W.); is described in Lord Byron’s Voyage in the Blonde as formed of a flat coral-rock, with no trees…’ – but the work is monumental and impressive.
However, despite the clarity of his reasoning and the mass of his evidence, not everyone was convinced. Towards the end of his life, feeling frustrated by the doubters, Darwin wished ‘that some doubly rich millionaire would take it into his head to have borings made in some of the Pacific and Indian [Ocean] Atolls’. He thought that borings to a depth of 500 or 600 feet (152-183 metres) would be sufficient. And if it turned out that he had been wrong in his ideas, then ‘the sooner I am knocked on the head and annihilated so much the better.’
A few months after wishing for annihilation should he be proved wrong, Darwin published his study of earthworms. At the time, the book was wildly popular, selling 3,500 copies within the first month. Today, though, it is little read, and often dismissed as the eccentric afterthought of a great man whose life was drawing to a close.
But it wasn’t an afterthought. True to form, Darwin had studied earthworms for decades. In November 1837, just over a year after returning from his voyage around the world, he gave a brief speech about the animals at the Geological Society of London and, a couple of years later, he published a short paper about them. In this paper, Darwin described several fields that he had visited with his uncle. Each of the fields had, some years previously (though no one could remember exactly when), been covered with a superficial layer of materials such as cinders. But, in each case, the layer had mysteriously disappeared. When Darwin dug holes in the fields, he discovered that the overlaid materials were now at some depth below the surface. His uncle speculated that this was due to the activities of earthworms. As they burrowed through the soil, the worms were, his uncle suggested, acting as slow-motion ploughs. Altogether, the observations were so interesting that William Buckland, an eminent geologist of the day, suggested that Darwin had identified ‘a new Geological Power’.
_in_Kent._Wood_engra_Wellcome_V0018691.jpg?width=3840&quality=75&format=auto)
As their family began to expand, Darwin and his wife decided they wanted to move out of London. Accordingly, in August 1842, a few months after his book on reefs was published, the Darwins bought a house in the country; it came with some land. Soon after moving in, he set aside some of that land to test his uncle’s hypothesis. From the outset, this was a long-term project: Darwin intended that the field should lie undisturbed for many years. In his science, as in his thoughts about the workings of the world, he embraced a long, slow view.
Earthworms burrow through soil by eating it; they also nibble on organic matter such as dead leaves. To defecate, they generally come to the surface, where they eject, as Darwin put it, ‘little intestine-shaped heaps’ known as castings. On the basis of his conversations with his uncle, Darwin suspected the worms of tilling the soil, bringing fine particles from deep in the ground up to the surface.
Darwin embarked on experiments to see how worms perceive the world. Were they creatures of tastes?
As a result of these repeated actions, he thought, the soil would be slowly turned over and mixed. New soil would be raised up, while objects at the surface would become dusted with earth and, as the years went by, would gradually become buried. To measure how long such a burial might take, in 1842, just before Christmas, Darwin had lumps of broken chalk spread on top of the field that he had set aside. Twenty-nine years later, he had a trench dug across the field. The chalk now formed a line through the soil, roughly 18 centimetres (7 inches) below the surface. From this, Darwin calculated that in each of the intervening years the worms had covered the ground with a layer of topsoil that was, on average, 0.56 centimetres (0.22 inches) thick. Something similar happened to a row of flagstones that, in 1843, he’d had placed to make a path across his garden lawn. ‘During several years the path was weeded and swept; but ultimately the weeds and worms prevailed, and the gardener ceased to sweep.’ The stones had gradually disappeared beneath the grass.
Never one to settle for a single piece of evidence, Darwin did not stop there. Once again, he mustered an enormous mass of material, from as many sources as possible, to support his claims and overwhelm potential objections. He embarked on experiments to see how worms perceive the world. Were they creatures of tastes, with active preferences? Yes, they like to nibble on cabbage leaves, but disdain thyme and sage. Moreover, leaves did not only serve as food. Darwin observed that earthworms often line their burrows with leaves, perhaps ‘to prevent their bodies from coming into close contact with the cold damp earth’. He also compiled huge numbers of observations from others, enlisting the help of four of his sons, as well as correspondents in places as diverse as India, Australia, Brazil and Venezuela.
Much of this work was unglamorous, consisting as it did of collecting and weighing those little intestine-shaped heaps of excreted soil. One of the most valiant efforts was made by his niece, Lucy Caroline Wedgwood. For a year, on a near-daily basis, she collected and weighed earthworm castings from two designated plots, each just under a square metre in size. On the less productive plot, the worms brought up just short of 2 kilogrammes of soil per square metre per year, which doesn’t sound like much. But scale up these numbers across space and time, and the results become impressive. Darwin estimated that worms move between 18.98 and 45.49 tonnes per hectare per year (7.56 to 18.12 tons per acre per year), depending on where they live. He reckoned that, considering the worm-friendly areas of England and Scotland together, earthworms would move more than 325 million million tonnes – in today’s parlance, that’s 325 trillion tonnes – of earth over the course of a million years.
If worms bury bits of chalk, Darwin reckoned that they would also bury other objects dropped on the ground – coins, gold jewellery, ancient tools. Nor was that the end of their powers. By analogy with the sinking flagstones in his garden, he suspected that worms could cause the burial of ancient ruins. By burrowing away below, worms would cause buildings to subside and sink into the soil; and by bringing soil to the surface, he thought that they would gradually cause the ruins to become covered up. And so off he went to find out.
Picture a hot day in August 1877. Darwin, by now, is an old man, the leaping pole long since discarded. He has a long white beard that makes him look vaguely like an Old Testament prophet or, as one of his eulogists would write, ‘an ancient philosopher’. He has travelled some distance from home to attend the excavation of the ruins of a Roman villa discovered beneath a field in Surrey.
On each of several mornings after the villa’s atrium had been excavated and swept of soil, Darwin knelt down to inspect the tiled floor – and found several little heaps of soil left by worms. The worms, it turned out, had come up through small gaps between the tiles. By snatching away the fresh castings, Darwin even managed to surprise several worms in the act of retreating into their burrows. From what he observed at the site, Darwin concluded that worms had been the chief agents of burial. ‘Archaeologists are probably not aware how much they owe to worms for the preservation of many ancient objects,’ he declared.
Taking his results together, Darwin showed that earthworms have several important effects. The animals do not just mix the soil by bringing deeper material up to the surface. By munching on fallen leaves, and by pulling those leaves down into their burrows, they also create new soil and enrich it with a nutritious compost. In addition, as earthworms eat their way through the soil, they grind it into smaller particles, breaking up small stones and milling the soil to a finer consistency. Earthworm burrows – which, in some places, can be well over a metre deep – also serve as channels that irrigate and aerate the soil, and make it easier for plants to send down their roots.
Over time, these activities transform the landscape. Worm castings don’t always stay put: rain, wind and gravity conspire to shift some of the soil that the worms upheave, tending to send it downhill. By measuring worm castings before and after wind and rain on slopes of different steepness, Darwin showed that, each year, some fraction of the soil excreted by worms flows downhill. While, from one year to the next, this would be an imperceptible creep, over many centuries, it adds up. Or, as Darwin put it:
When we behold a wide, turf-covered expanse, we should remember that its smoothness, on which so much of its beauty depends, is mainly due to all the inequalities having been slowly levelled by worms. It is a marvellous reflection that the whole of the superficial mould over any such expanse has passed, and will again pass, every few years through the bodies of worms. The plough is one of the most ancient and most valuable of man’s inventions; but long before he existed the land was in fact regularly ploughed, and still continues to be thus ploughed by earthworms.
Worms might appear insignificant, but because there are so many of them, little by little, they sculpt the contours of the world.
When the corals of Enewetak first started growing, Mount Everest was still millions of years in the future
More than a decade after Darwin’s death in 1882, ‘a doubly rich millionaire’ duly appeared, eager to discover whether, somewhere beneath each atoll, you do indeed find the sunken remnants of a volcano. To test the idea, in 1896 the millionaire – in the guise of the Royal Society of London – dispatched an expedition to the atoll of Funafuti, in the South Pacific Ocean. After two years of struggles and false starts, the team managed to drill to a depth of 340 metres (1,114 feet) – a remarkable accomplishment given that they were working with a drill powered by a coal-fired steam engine. (All the coal, which amounted to well over 140 tonnes, had to be shipped in.) But despite having drilled so deep – rather deeper, indeed, than the 180 metres (600 feet) that Darwin had suggested – they found nothing but the remnants of corals and other reef-building lifeforms. In the 1930s, the Japanese sent an expedition to Kitadaitōjima, an island once known to Europeans as North Borodino, in the Philippine Sea. This team drilled down to 431 metres (1,416 feet); but, again, they found only life-built limestones and shells. In both cases, the reef-builders had clearly grown in shallow waters, a finding consistent with the idea of subsidence; but clinching proof of Darwin’s hypothesis remained elusive.
Enter a new, richer millionaire, in the sinister form of Uncle Sam. In the 1940s and ’50s, the United States government tested dozens of nuclear weapons in the Marshall Islands, a cluster of atolls that lie in a remote part of the Pacific a little to the north of the equator. This brought a host of scientists – geologists, biologists, oceanographers – to study the area. In 1952, a team of geologists drilled deep into Enewetak Atoll. At 1,283 metres (4,208 feet), they struck basalt. Volcanic rock. Darwin was right.
Fossils found in the limestones just above the basalt show that the reefs of Enewetak Atoll began growing more than 50 million years ago. Back then, the planet was another world. The Atlantic Ocean was far narrower than it is today, much of Antarctica was clad in forest, and the Himalayan Mountains had yet to be upheaved. It is strange to think that when the corals of Enewetak first started growing, Mount Everest, the highest peak of the world today, was still millions of years in the future.
The growth of the Enewetak corals has not been continuous: at times either the sea level fell, or the atoll rose, exposing the corals to the air and the rain, killing the lifeforms and allowing the rock above the surface of the sea to be eroded away. Even so, today, the volume of limestone, of life-built rock, that makes up the atoll is estimated to be more than 1,000 cubic kilometres – or more than 250 cubic miles. On one atoll. That’s equivalent to building the Great Pyramid of Giza more than 400,000 times. If I could travel back in time to meet Darwin, that’s something I would want to tell him. That, and the fact that the Great Pyramid itself was constructed chiefly from limestones built by lifeforms. Not a reef, in this case, but a rock built from gigantic accumulations of the shells of nummulites – single-celled lifeforms that lived and died in vast numbers soon after corals first began to grow on Enewetak. I like to imagine that he would have been delighted.
But if Darwin was right about corals, he was, in one fundamental respect, wrong about worms. At the end of his book, he remarks: ‘It may be doubted whether there are many other animals which have played so important a part in the history of the world, as have these lowly organised creatures.’ In this, however, he profoundly underestimated the scale of the changes wrought by other lifeforms.
First off, the tilling processes that Darwin described are not limited to earthworms, with different burrowing animals having effects at different scales. Ants, for example, tend to bring up the finest grains of sand or soil. In Berlin, where I live, you often see small piles of fine sand heaped along the edges of cobblestones, and if you look closely, you will often see ants hard at work. Although the grains are typically brought up a few at a time, in places the ants are so abundant that they will shift many tonnes of soil per hectare per year. In one study that was directly inspired by Darwin’s work on earthworms – Geologic Work of Ants in Tropical America (1910) – the author, John Casper Branner, estimated that ants in Brazil were responsible for moving considerably more soil per hectare each year than earthworms in England. Meanwhile, larger burrowing animals such as badgers excavate huge mounds of earth, creating hillocks that can last for centuries, to say nothing of the earth-shifting activities of bandicoots, beavers, chipmunks, gophers, meerkats, mice and moles, or of burrowing birds and burying beetles.
And it’s not just on land. As you walk to the sea across that part of a beach or mudflat that’s covered by waves when the tide is high, but exposed when the tide is low, you may see tiny crabs, no bigger than your fingernail, excavating burrows one armful of sand at a time. Don a mask and look beneath the waves, and you’ll find an incredible variety of animals digging burrows.
As oxygen is reactive stuff, its arrival led to a proliferation of new minerals. Earth began to rust
Moreover, burrowing animals are not the only lifeforms to have a substantial planetary impact. Far from it. Before the evolution of the first trees, around 400 million years ago, rivers were far less likely to boast meanders and oxbows and other features that allow them to dawdle their way to the sea. Plants have also contributed a great increase in mud. Ancient reefs and banks of shells have not only left a legacy of enormous piles of limestones, they also have altered the shapes of mountains: as rocks go, limestones are relatively malleable, so when ancient reefs and banks of shells are crushed and cooked as mountain chains are upheaved, the presence of limestones will affect the way the mountains fold. The more limestones, the more folds. Limestones so treated will also, often, become crushed and cooked into marble. Many of the world’s greatest sculptures and monuments have been created from rock built by life and then transformed by Earth as it builds mountains. Next time you look at Michelangelo’s David, remember that it was built from marble formed from crushed life-rocks, as were many of the grandest buildings and structures of ancient Rome.

Long before animals or plants evolved, moreover, the planet was being sculpted by much smaller lifeforms – bacteria and archaea. (Of these, bacteria are far better known. That’s because archaea were not identified until the 1970s. Seen through a microscope, archaea superficially resemble bacteria – both are small and tend to take shapes such as rods or spheres. So it was not until the development of molecular tools that it became clear that the two groups are distinct.) I will not enumerate all the effects these lifeforms have had, as it would take too long. Instead, I will give just two examples of their impacts. First, certain species of archaea are responsible for the biological generation of methane, a greenhouse gas, which warms the climate. Second, no conversation about the impacts of lifeforms upon the planet would be complete without mention of the cyanobacteria. These lifeforms, formerly known as blue-green algae, are, in my view, the most important lifeforms in the history of the planet. At the time they evolved, more than 2.3 billion years ago, Earth had no oxygen molecules in the air to speak of. Instead, all the oxygen atoms were tied up in the water and the rocks. Cyanobacteria evolved to use the radiant energy of the Sun to split water molecules apart, a process that roughly halfway through the history of Earth would result in an atmosphere that contained oxygen molecules.
Back then, the atmospheric oxygen would not have been adequate to support you or me. Yet its very appearance had several transformative effects. As oxygen is reactive stuff, its arrival led to a proliferation of new minerals. Indeed, Earth began to rust. At the same time, the advent of oxygen led to the creation of an ozone layer, which protects the planet’s surface from the most harmful rays of the Sun. The presence of this layer fundamentally changed the circumstances in which lifeforms on land subsequently evolved. And since the colour of the sky is a consequence of the composition of the atmosphere, lifeforms have also reached out and painted the heavens above.
I could go on. But rather than make a long list of all the changes that lifeforms have wrought, I would prefer to present the effects differently, to go back to the idea of the Never-Life Earth, and describe what the planet would be like today if no lifeforms had ever been here.
What if this planet had never come alive? What if no living beings had ever breathed or swum, burrowed or flown here? How would Earth be different now, a little more than 4.5 billion years into its history?
At first, the answer seems simple: just remove the lifeforms. Strip away the trees, the grass, the bushes. Scrape the moss and lichens off the rocks. Vanish the animals, annihilate the mushrooms, disappear the microbes. Silence the birds, frogs, cicadas. Empty the oceans of fish, jellyfish, octopus, whales, crabs; skim the ponds of slicks and scums. And then, with all the lifeforms gone, you’re done. Right?
Not so fast. Removing the lifeforms is just the start. To create an Earth that had never had life, you also have to take away the detritus of life – all the things humans have made, of course, but also the fallen leaves, the pearls, the spiders’ webs. You have to sieve the pollen from the wind, and empty the soil of spores and seeds. You have to dig out the guano from caves where bats roost, and from islands where seabirds nest. You have to fill in the wallows where sparrows bathe in the dust, and repair the pits on the seafloor where whales rootle for food. You have to flatten the mole hills, ant nests and termite mounds, and bust the beavers’ dams.
Once you’d dealt with the life of today, you’d have to turn your attention to the past. You’d have to remove the fossils – the prodigious piles of bones and shells that litter the planet, the vast petrified forests, the ancient reefs. You’d have to erase the footprints of dinosaurs, fill in the burrows of long-dead worms, and rub out the imprints of fallen flowers. You’d have to get rid of the amber, siphon off the oil, and shovel away great mountains of coal.
And still you would not be done. You’d have to undo transformations wrought by life that, at first, appear to have nothing to do with it. You’d have to get rid of all the chalk, much of the marble, some of the diamonds. Many types of minerals – more than half – would have to go too. You’d have to suck oxygen from the air, and take away the ozone layer. You’d have to extinguish the fires, clean away the charcoal, and scrub the soot from the rocks. You’d have to sweep the topsoil from the ground, sharpen the edges of the mountains, and straighten the meanders in the rivers.
Humans are affecting more dimensions of the life-Earth system than any lifeform has previously
More speculatively, the sky might not be blue. The Moon might be at a different distance from Earth, and the length of the day might not be the same. The climate would be hotter and more arid.
In short, the Never-Life Earth would not be the Earth of today, just without the green. It would be profoundly different: an alien planet. A human, or some other animal, magicked there would be killed in an instant, overcome by suffocating air and lethal levels of radiation.
It would be a planet not merely lifeless, but deadly.
As more and more people crowd into cities around the world, more and more of them say they feel disconnected from nature. At one event I went to, ‘connection’ to nature was being promoted through virtual reality; individuals sat indoors, alone in their masks, removed from actual reality, watching immersive films. And this was to celebrate the birthday of a world-renowned ecologist. So it is an irony that, considered together, humans are more connected to nature now than any species has ever been before. Humans are not the first species to change Earth. But through our activities, humans are affecting more dimensions of the life-Earth system than any lifeform has previously – and the impacts are accumulating more rapidly. Far more rapidly. The effects that we are having upon the planet are unprecedented in their scope, and unprecedented in their speed.
Again, one plastic bag does not make a rubbish dump. But do a Darwin, and sum up the cumulative impacts, add together all the plastic bags that humans have ever made, and you will find that the effect is dramatic. Similar summings-up apply to the impacts of airplane flights and car journeys, to paper cups and plastic straws, to the use of fertiliser, or to any other aspect of human activity that you care to name. But if we are distinguished by the scale and speed of our impacts, we are also distinguished by our awareness. As far as anyone can tell, humans are the only lifeforms that have ever been able to study the world and know what we do – and that brings opportunity. Perhaps, together, we can reduce our impacts and develop a new ethos of planetary care.
I would like to end on a personal note. Contemplating the entwined histories of life and Earth has changed the way I see the world. It has heightened my interest in all lifeforms, no matter how humble, and it has enhanced my sense of connection to the air, the water, the rocks. I find it majestic to think that the air I breathe and the rocks I tread have been shaped by countless lifeforms that lived and died ages ago. To me, this view of life and Earth is poignant, beautiful, and grand.
This piece is dedicated to Bob Hazen, whose work inspired me to begin studying the histories of life and Earth, and to David Catling, for his generosity and grace in helping me to understand some of the other planets that Earth has been.