In a dramatic scene that some readers will recall from childhood, the mongoose Riki-tikki-tavi encounters the villainous cobra Nag for the first time. The story’s author, Rudyard Kipling, meticulously describes the false eyes that some cobras have on the back of their hoods:
[I]nch by inch out of the grass rose up the head and spread hood of Nag, the big black cobra, and he was five feet long from tongue to tail … he spread out his hood more than ever, and Rikki-tikki saw the spectacle-mark on the back of it that looks exactly like the eye part of a hook-and-eye fastening…
The cobra’s false eyes are a likely example of warning signals: adaptations that prey use to tell potential predators: ‘If you attack me, I promise things will go badly for you.’ Both predator and prey benefit from this message: the prey has the chance to avoid an attack, and the predator can make an informed decision about whether or not to risk pouncing on a well-defended meal.
The function of the cobra’s hood is intuitive, and squares nicely with how we often expect evolution to work. In fact, it’s so beautifully simple that it raises the question of why warning signals should evolve into such complex forms in the first place. A cobra could probably warn off predators with a much simpler design that’s less trouble to manufacture than its wide hood, eerily recognisable eyes, and even a definite ‘nose’ between the eyes. Instead, the cobra could have opted for just a bit of red around the neck, which other venomous snakes possess. After all, the function of warning signals depends on making it obvious to the predator that this potential piece of prey is not to be meddled with. So, anything that appears striking and unambiguous should do the trick – and if there are fewer complexities that could go awry during development, or be misinterpreted, so much the better. Why would natural selection favour the assemblage of the complex genetic machinery needed for false eyes, or for that matter, the thousands of other complex signals we find in nature?
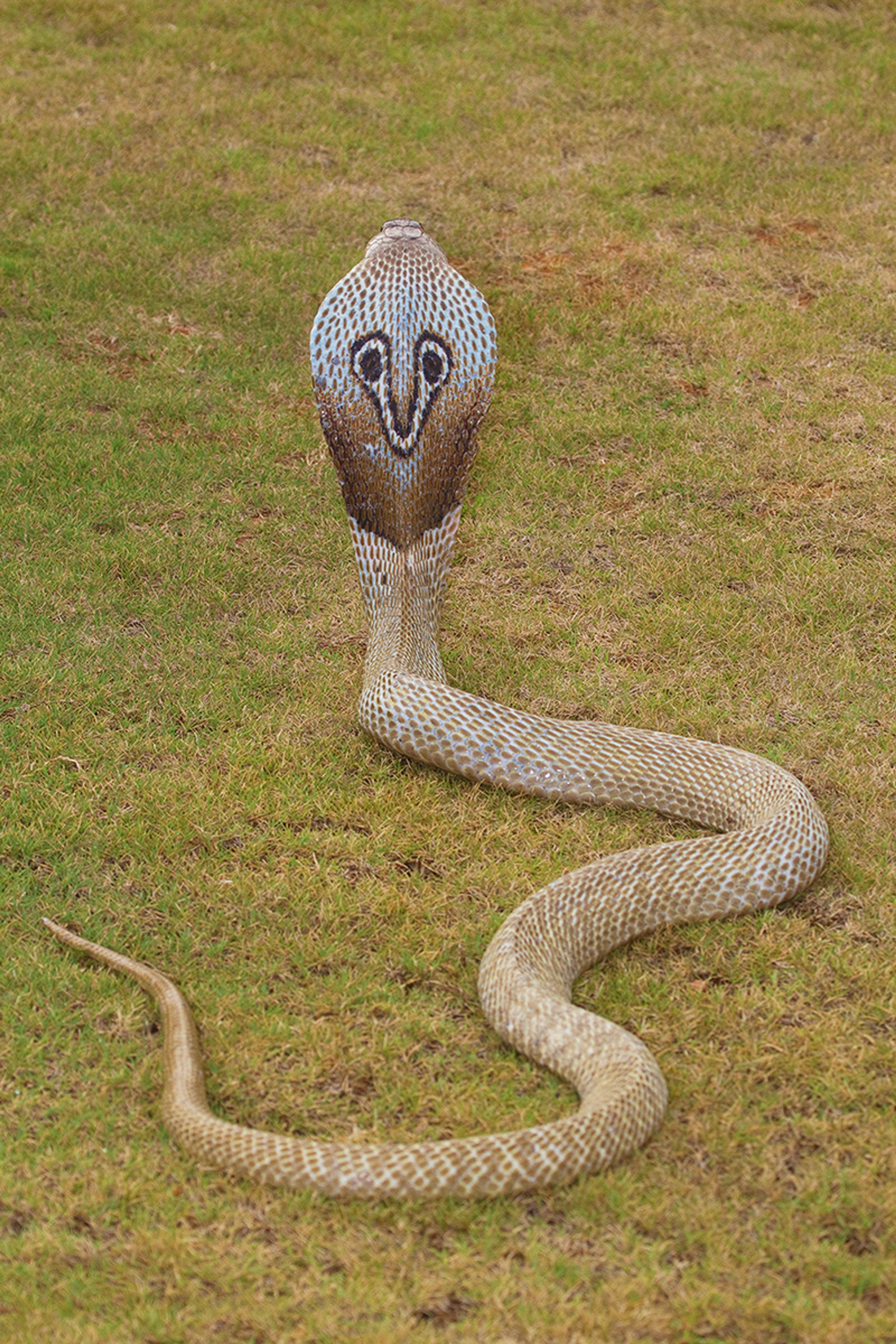
Photo courtesy Krushnamegh Kunte
Some of the most convincing false eyes are found on the spectacled cobra of India, Naja naja. India is a marvellous region for reptiles and amphibians. Its fauna includes legions of venomous elapids (the family that contains cobras), along with various vipers, constricting pythons, skinny vine snakes, aquatic keelbacks, four of the world’s ‘flying’ snakes, vermicious caecilians, monitor lizards, the whimsical yet hopeless-looking balloon frog, and unorthodox crocodilians such as the gharial.
One might think that the cobra’s eyes originated by chance from among all this wild diversity, and that selection had no role in their evolution. This is a tempting explanation but seems unlikely, since the pattern is highly regular, and the eyes’ position on the hood makes it particularly visible. However, we might make headway by considering the cobra’s number-one predator: humans.
In India, humans frequently encounter snakes. Venomous snakebites are a major threat to public health, leading to approximately 50,000 fatalities every year. Humans have an understandably antagonistic relationship with serpents, often killing them upon encounter. At the same time, however, snakes occupy a vaunted place in some aspects of Buddhism and Hinduism. A cobra allegedly spread its hood to shelter Siddhartha Buddha from the rain while he meditated under a tree and, in Hindu mythology, the Naga are benevolent serpent folk. One of them supports Narayana, the supreme deity, in the cosmic ocean while he holds up the world.
It’s possible that these mythological gestures of goodwill have had real evolutionary consequences for existing snakes. In his book Snakes: The Evolution of Mystery in Nature (1997), the herpetologist Harry Greene speculates that humans’ sympathetic perception of snakes in places where they’re associated with myths and legends might have selected for the origin of cobra eyes. Those cobras with proto-eyes were most likely to elicit our sympathy from ancient myths, and so to escape the scythe. Across the generations, this process of culling the least sympathetic cobras could have given rise to the spectacled pattern that we know today. To be fair, whether or not myth truly shaped human aesthetics so that we would unconsciously breed cobras for eyespots hasn’t been tested empirically, which would be very hard to do. Yet it still illustrates a critical point: although natural selection must favour the evolution of warning signals if they’re to evolve, what any given signal looks like depends on the eye of the beholder.
Another branch of the venomous elapid tribe of snakes has enjoyed great success in the Americas. In Arizona, one moonless night after a monsoon rain, I encountered one crawling boldly across the trail: it was a coral snake, so called because of the bright rings, usually red, that encircle the bodies of most species. They are perhaps the most beautiful snakes in existence. When I ran to observe this individual, it calmly finished its crossing and curled up in front of a small pile of rocks to face me, its rings of carmine, white and black glinting in my headlamp. The pattern was exquisite, a complex string of urgent, unpronounceable warnings. Each unit of its tricolour motif occupied a particular proportion of the snake’s body, presented in a particular order, that repeated itself 11 times from head to tail with uncanny precision. Was all this really necessary to deter predators?
During my doctoral studies, I studied coral snakes to try to answer the question of just how precise a warning signal needs to be for it to work. In North Carolina, one species of coral snake lives alongside what we call a Batesian mimic: a harmless species that has evolved to resemble a better-defended one. Batesian mimics have ‘warning signals’, but no accompanying defences. The mimic in question was the scarlet kingsnake, which is completely nonvenomous. Interestingly, its coloured rings are arranged in a different order from the coral snake’s – instead of deadly yellow-black-yellow-red, the kingsnake has black-yellow-black-red. This is the basis of the children’s rhyme ‘Red touch yellow, kill a fellow; red touch black, you’re okay, Jack!’ So its mimicry can fool only a predator such as a hawk or a coyote that doesn’t know this simple difference between them. In nature, predator numbers are often limited by starvation, so it would be surprising if they couldn’t learn to discriminate tasty kingsnakes from deadly coral snakes. Therefore, it seemed likely to me that some constraint in development prevented the scarlet kingsnake from evolving better mimicry: probably, if the mimicry were more precise, the scarlet kingsnake would be attacked less by predators.
The biologist David Pfennig and I tested this prediction by making hundreds of little clay snakes and putting them out into the longleaf pine forests of North Carolina. After allowing wild predators to attack the snakes, I analysed which colour patterns were best-protected. To my surprise, the ring order had no effect on whether or not the clay snakes were attacked: only the ratio of red to black on the snake mattered. So the precision of the mimicry was entirely down to the perception of the predator, who chose their meals based only on a coarse resemblance. Although coral snakes have what are in many ways elaborate patterns, some of this complexity probably came down to chance – the colour pattern of whichever ancestral elapid snake happened to emigrate from Asia to give rise to New World coral snakes.
Why does such perceptual sloppiness on the part of predators persist? Shouldn’t selection lead to predators with keener powers of detection? In answer, consider the account of a red-tailed hawk falling dead from the sky, a coral snake in its talons. Coral snakes are deadly and give predators no real opportunity to learn to avoid them. Perhaps because the consequences of making a mistake are so severe, then, evolution instead favours generalised avoidance. It’s vital to be correct on the first encounter. Indeed, for many years, some members of the scientific community doubted that coral snake colour patterns could be warning signals at all, because they presumed that learning about coral snake venom meant a quick exit from the predator population.
When she painted the dowel with rings of coral-snake colours, the birds fled, crying in distress
In the 1970s, Susan M Smith tested the proposition that predators could avoid deadly prey. She hand-reared two species of birds that were native to Costa Rica, where they co-occur with coral snakes. When Smith obtained the young birds, they had never observed a coral snake, and she made sure that they had experienced only non-threatening food during development. After her nestling birds had fledged, Smith trained them to peck at a long, snake-like wooden dowel in their cages to receive food. After the birds had mastered this task, Smith painted the dowel in different colours. She carefully recorded how the birds responded to changes in the colour pattern on the dowel. Some of the colours that she tried were drab, like the camouflaged vipers that inhabit the fields and forests of Costa Rica. Others had coral-snake colours but arranged in long stripes instead of the rings on coral snakes.
Smith found that none of these treatments affected the eagerness with which birds pecked at the dowel to receive their victuals. However, when she painted the dowel with coral-snake colours that were arranged into rings, all the birds lost their composure and fled to the opposite ends of their cages, where they cried in obvious distress. None so much as pecked at the dowel.
It seems, then, that the avoidance of warning signals didn’t have to be learned – predators could evolve to innately fear them. What was particularly surprising in Smith’s work was the specificity of the birds’ innate aversion. The long, snaky dowel, the use of coral-snake colours and the arrangement into rings were all necessary to deter birds from pecking. Somehow, all that information – and what to do with it – was inscribed into the genes of birds that have a long evolutionary history with coral snakes.
Amazing though Smith’s results were, there were limits to birds’ inbuilt powers of recognition. The rings didn’t have to be in the precise order in which they would be found on a real coral snake, and not all the colours needed to be present. Red rings, and another contrasting colour such as yellow presented on a snake-like object, were sufficient to make the birds stay away.
There’s a direct relationship between Smith’s behavioural observations, and field experiments with clay replicas. Taken together, they’re strong evidence that the combination of colours and patterns that predators have evolved to avoid generally match those that prey deploy for protection in the wild. Yet it seems unlikely that the future will bring us predators that can more finely discriminate coral snakes from their mimics. Once a predator has evolved a broad tendency to avoid anything that generally looks like a coral snake, it becomes less likely to attack those sorts of objects or creatures – so any selection pressure upon predators to avoid other aspects of coral snakes will be very weak. Yet when we look at a coral snake, we might not only marvel at how its colour and pattern have been shaped by predator perception but also, in turn, how the coral snake has shaped the neurobiology of some of its enemies.
In the coevolutionary dance between predator and prey, warning signals are an elegant step. With seeming ingenuity that’s driven by blind natural selection, they reduce the possibility that an individual prey will be attacked. Direct measurements of predator behaviour, such as those done by Smith, show that possessing a warning signal can confer an overwhelming advantage over prey that don’t have them. Warning signals also seem to confer an advantage in some natural contexts, such as my experiments with coral snakes and a single species of Batesian mimic.
It would seem that we should find warning signals everywhere. Yet when we look across the whole of the animal kingdom, strangely, they’re relatively scarce. Among groups that have been examined systematically, only 5 per cent of amphibians, and 5 per cent of lepidopteran larvae (the young of moths and butterflies) are warning-coloured. Within the mammals, skunks and some weasel family members can exhibit warning signals, but other examples are rare.
Some researchers have systematically examined the success of warning signals across the tree of life. Sophisticated algorithms can take information about species that currently exist and trace their evolutionary route backwards along the branches of the tree to estimate what they looked like in the past. In doing this sort of analysis, it’s possible to examine how often species change from one state to another. For example, they can change from being cryptic (camouflaged) to having warning signals – from operating by stealth, to telling their predators to back off.
When Alison Davis Rabosky and her colleagues performed one of these analyses on snakes, they found that in past aeons, there were many ancient species of nonvenomous snakes that switched from camouflage to looking like coral snakes (that is to say, they became Batesian mimics). But they also found that ancient species of Batesian mimics were just as likely to switch back to being camouflaged. This suggests that Batesian mimics are limited in numbers, and whatever creates this limit can favour camouflage instead of mimicry.
Red-winged blackbirds ordinarily avoid white butterflies, but resort to them in times of need
Kevin Arbuckle and Mike Speed conducted a separate analysis of all warning colours (including all warning-coloured species, most of which had defences). They examined the tree of life for amphibians, a completely different group of animals. Warning colours in amphibians tended to increase the rate at which new species formed – so that the evolution of warning colours tended to beget more species with warning colours. However, at the same time, warning colours were lost very quickly, because species with warning colours often switched back to camouflage. This means that not only does selection sometimes favour switching from being a Batesian mimic to camouflage, but it can also favour switching from honest warning colours to camouflage.
These dynamics reveal that the evolutionary success of a warning signal often sows the seeds of its own failure. Such facts are at odds with the prevailing superiority of warning signals that we find with simple experiments in the lab, and from experiments in some natural ecosystems. They suggest that there is an aspect of biology we’re failing to account for, something that works against the success of warning-coloured species.
We don’t yet know what that ‘something’ is, but predators’ perception might be an important component. Perceiving your prey as palatable or unpalatable is a fickle thing: when you’re full and well-fed, a butterfly with a bitter taste is easy to disdain. But when you’re ravenous, and your babies have hungry maws to fill, your perception might transform that same butterfly into a delicious meal on wings. Once predators exhaust other food, they can shift their behaviour, changing those bright colours from warning signals into highly visible targets. Such a shift can occur among red-winged blackbirds, which ordinarily avoid white butterflies, but resort to them in times of need. If the availability of tasty, alternative prey changes consistently over long time periods, it could cause defended species to adapt by losing their warning signals, or making themselves scarce. When Batesian mimics evolve to resemble warning-coloured species, theory predicts that they will tend to make the problem worse: the presence of liars will make predators less likely to heed warning signals. And once warning signals lose their meaning, they can quickly go extinct.
The number of kinds of prey available, and the abundance of Batesian mimics, might help us explain the repeated appearance and disappearance of warning signals from the tree of life. We can’t make experimental tests when dealing with phenomena that span millions of years, so studies must rely on indirect methods of inference. The best way to do this is to consider not just how predators learn to avoid prey with particular warning signals, but how the community of prey they choose within also affects their decisions. And this means shifting our own perspective: instead of focusing on a single, idealised pair of predator and prey species that reciprocally adapt, we must ask how the natural milieu in which they coexist shapes their relationship.
I began this essay with the cobra, with eyespots that emblazon themselves in our minds, and have perhaps even evolved because they have just this effect. Many of the most entrancing animals in nature – such as brightly coloured butterflies, coral snakes, fiery newts, black-and-yellow wasps, ladybirds and honey badgers – look the way they do because they elicit avoidance from other animals in their environments. We find them striking because our own perceptual systems are not so different from those of other visually oriented predators. Lynne A Isbell, an anthropologist, has even hypothesised that our primate brains evolved their visual processing capacities in response to the threat posed by snakes. Whether or not that’s true, we are undoubtedly part of a web of signal and perception. It includes far more than warning signals alone, or the relationships between predators and prey. Perceptual biases have been uncovered in many sexually selected signals, from swordfish tails to frog calls. The networks of pollination that sustain our ecosystems are linked according to pollinator preferences for floral signals in particular arrangements of colour, shape and scent. So it’s no exaggeration to say that species are structured not only into food webs, but also into webs of information.
Connections in this web can arise via many evolutionary paths. Signals evolve in response to the perception of specific receivers. In some cases, a receiver’s perception might also coevolve with the signal, intertwining the two. Other animals that originally had nothing to do with the initial evolution of a warning signal also get caught up in the web. For example, we don’t know whether the species of birds that Smith worked with were the predators that led to the origin of coral snake rings. They might have been innocent bystanders that simply evolved to avoid what is dangerous in their environments. Nevertheless, now that those birds avoid coral snake rings, they probably contribute to at least some selection that maintains the coral snakes’ appearance. Other additions to the web of communication have the opposite effect, potentially weakening it: Batesian mimics take advantage of the safe space created by the warning signal, but at the same time corrupt it. They might ultimately cause warning signals to revert to camouflage.
All these species are tangled together with threads of information, each driving the evolution of the others. So far, we have explored only a few threads. One of the promising future directions for understanding the whole tapestry is to use experiments to test how the ensemble of prey species influences how predators perceive warning signals. This will help us understand why, when we venture out into the forest, most of it is green, and many animals within it are hidden, nondescript or easy to ignore: why it is rare to find a brightly coloured, venomous snake, and why, when we do, it holds our attention rapt.