The Universe began as a strange sort of soup. Large galaxies hadn’t yet formed, and flying particles were hotter than the centres of stars in the Universe today. Of these, tiny particles known as quarks clumped together to form the building blocks of atoms: neutrons, protons and electrons. Through a process called cosmic inflation, the Universe expanded like a balloon, smoothing out the clumps.
It could be that the missing matter of the Universe – called dark matter by physicists – formed then, too. Dark matter is one of astronomy’s notorious mysteries. Evidence has grown over the past century that there must be something out there besides the stuff that makes up our tables, our planet, even ourselves. An early hint, in the 1970s, came from the astronomer Vera Rubin, who showed that stars at the edges of galaxies rotate faster than we’d expect from just the mass we can see through telescopes. Something else heavy had to be there, exerting enough gravity to make the galaxies spin. Since the 1980s, astronomers have agreed: dark matter must exist to explain that ‘missing mass’ in our observations, but we can’t see it since it doesn’t interact with light in the typical ways. Our current best estimates show that there should be about five times as much dark matter as ‘regular’ matter in the Universe. In fact, all the things we interact with in our daily lives make up less than 5 per cent of the matter in the Universe.
‘Dark matter makes up 25 per cent of the Universe and we have no idea what it is!’ says Leah Jenks, a theoretical physicist and PhD candidate at Brown University in Rhode Island. ‘So, in that sense, it is a hugely important open question in the field. I think it is also a fun problem to work on because there is a lot of room for creativity in trying to think of new ways we might be able to understand this mysterious missing matter.’
So far, we’ve had many ideas, but little evidence to confirm any of them. Physicists have spent a lot of their time looking for new particles to explain the missing mass. One of the most popular candidates, a collection of big particles called WIMPs, or weakly interacting massive particles, has recently fallen out of favour, since experiments haven’t been able to find evidence for them. Despite looking with particle accelerators, large underground particle-detecting chambers, and other experiments, the Universe has given us no signs that dark matter is one of these elusive particles. This has led scientists to look for other, extremely tiny, particles subject to strange quantum phenomena (known as ‘fuzzy dark matter’) or even changes to the fundamental laws of physics as we know them (an often-shunned theory known as ‘MOND’).
But with a dearth of evidence for any of these ideas, a dark horse theory has emerged. In those first seconds of the Universe, there might have been another ingredient in the primordial soup: black holes. These black holes from the very beginning of time, known as primordial black holes (PBHs), could still be lurking around today – and some scientists believe they could solve the problem of dark matter.
Black holes typically form from the deaths of the largest stars, where all the mass of a star collapses down to one extremely dense point. Their gravitational pull is so great that nothing, not even light, can escape. But in the first second of the Universe, stars didn’t yet exist – so how could black holes have formed back then? Pretty easily, calculations show, from the denser parts of the cosmic soup. The basic recipe, says Alexander Kusenko, professor of physics at the University of California, Los Angeles, is to ‘take a spoonful of the early Universe and squeeze it by 30 per cent.’
Kusenko and some others believe primordial black holes are now the most promising candidate for dark matter that we have. The real appeal of PBHs is their simplicity – unlike other options for dark matter, which require making theories to describe new particles, we already have evidence that black holes are real.
Primordial black holes aren’t a particularly new idea, though. Their story begins back in 1960s Russia, when two physicists – Yakov Zeldovich and Igor Novikov – first considered the possibility of extremely dense objects like black holes forming in the very early Universe. Their calculations showed that these dense regions would grow too fast and heat up the early Universe with their strong radiation, which decidedly conflicted with observations of the real Universe as we know it.
But in the 1970s, the British physicist Stephen Hawking and his graduate student Bernard Carr began looking deeper. Together, they wrote the first paper showing that primordial black holes might be a real possibility after all, finding a way around the problem of runaway growth. ‘That’s what put primordial black holes on the map again, since there was no reason to believe they couldn’t exist,’ says Carr, now emeritus professor of mathematics and astronomy at Queen Mary University of London.
Soon after, Hawking discovered what became known as Hawking radiation, which makes black holes slowly evaporate. For larger, star-sized black holes, the time it would take them to evaporate is far too long to be noticeable – longer than the time the Universe has existed. For small PBHs, however, we might be able to see it. A black hole about the mass of a mountain and the size of a proton, formed billions of years ago when the Universe began, should be showing the explosive end of its life right about now.
Since Carr and Hawking’s original theories in the 1970s, many scenarios have been proposed for how primordial black holes could form in the first fraction of a second of the Universe’s existence. In this long-ago era, the infant Universe was extremely hot, filled with high-energy particles and light, and behaved entirely unlike what we’re familiar with today. The key to making a primordial black hole is somehow making a small region of that hot early Universe slightly denser so that it can collapse into a black hole.
It turns out there are many ways that a part of the early Universe could become extra-dense. The simplest possibility is lumpiness here and there reveals ‘primordial inhomogeneities’. This is not too dissimilar from the fluctuations seen in the cosmic microwave background (CMB), the faint radiation we see from the moments the Universe became transparent a few hundred thousand years after the Big Bang. The CMB shows up in every direction we can point a telescope, but there are some minuscule fluctuations that only our most precise instruments can pick up. These fluctuations are essentially random, a result of the statistical nature of particles moving around in the early Universe. Another possibility is that inflation, the process that quickly expanded the early Universe just after it formed, created more fluctuations in density that could then go on to become black holes.
One model, which Carr is currently working on, proposes that primordial black holes formed at a time known as the QCD transition, an absolutely minuscule 100,000th of a second after the Big Bang. QCD stands for quantum chromodynamics, the branch of theoretical physics that deals with how particles interact via the strong nuclear force, which binds the nuclei of atoms together. The QCD transition, then, is when the Universe went from a soup of quarks and gluons – the components of protons and neutrons, and the glue that binds them together – to a soup of fully formed protons and neutrons. At this unique moment in the Universe’s history, it would be easier to make PBHs. You wouldn’t have to squeeze your spoonful of the Universe as hard to get it to collapse.
Kusenko, on the other hand, is working on a model that adds just one new bit of physics – a new kind of interaction between particles known as ‘Yukawa forces’ – to squeeze together enough matter to form just the right amount of primordial black holes to account for dark matter. Adding a new bit of physics is by no means unprecedented. In fact, that’s practically the job of the theoretical physicists working in this field.
Primordial black holes can wriggle their way into the centres of neutron stars to devour the entire neutron star
A whole host of other models rely on some of the most fantastical ideas in physics. One theory suggests that cosmic strings – cracks in the fabric of the early Universe – might sometimes loop around on each other to create black holes. Another theory proposes that ‘baby universes’ from the multiverse could appear in our own Universe as primordial black holes. If all these phenomena happened, but at slightly different times during the Universe’s first few seconds, the result would be various sizes of primordial black holes.
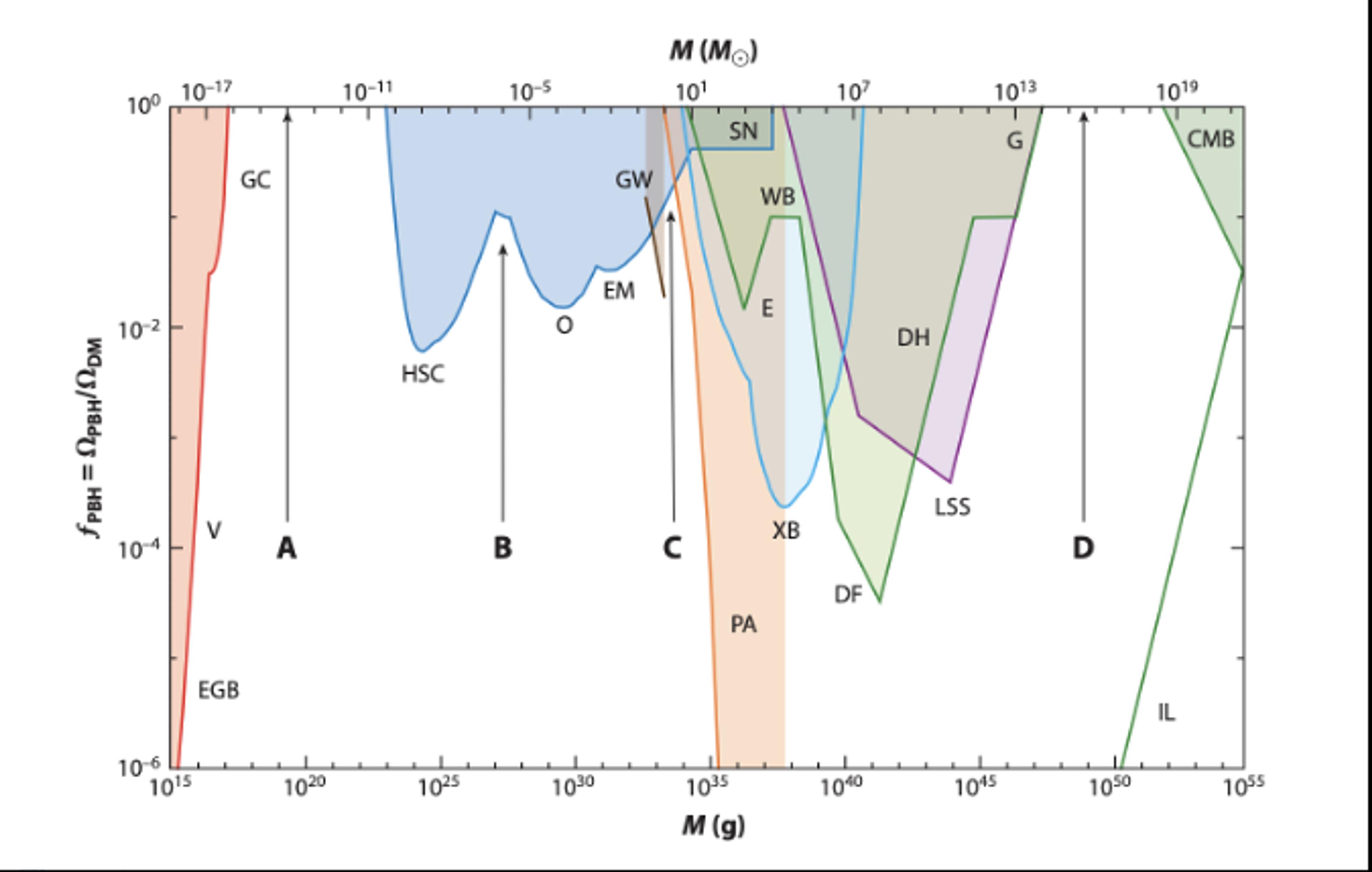
All the various types of primordial black holes we’ve ruled out: any region coloured in is where we do not see evidence for PBHs. The x-axis shows the mass of the black hole, and the y-axis shows what fraction of dark matter those PBHs can explain (where 10^0 is 100 per cent). Image: Carr and Kuhnel 2020
For decades, a small handful of physicists have considered these theories and others with a focus on how they would affect the early Universe if they panned out to be real. In fact, since primordial black holes would have such outsized influence, physicists have come up with numerous ways to prove or disprove they are real.
The very smallest PBHs are immediately ruled out as a dark matter candidate by Hawking radiation, since they wouldn’t live long enough to still exist now. Black holes about the size of a proton, on the other hand, could survive until the present day, but then explosively evaporate – and we just don’t see anything like that, so they’re ruled out, too. Even if primordial black holes weren’t glowing from Hawking radiation, they would be emitting light as they eat up matter, heating up the area around them. By looking at the history of the Universe’s temperature, scientists can set another limit on primordial black holes.
In our modern Universe, PBHs in a galaxy could be randomly flying about, disrupting the orbits of stars with their gravity and nudging them slightly out of place in ways we can observe. Scientists have also predicted that some PBHs will settle into the centre of the galaxy, like sand falling into a funnel. Measuring the amount of mass at the Milky Way’s centre could tell us the maximum amount of PBHs that could be lurking there.
One of the most dramatic ways primordial black holes can interact with matter, though, is by colliding with other objects in the Universe, like stars and planets. Primordial black holes can wriggle their way into the centres of neutron stars, the dense leftovers from a dead massive star’s core. Once they finally reached the centre, they would quickly devour the entire neutron star from inside, destroying it in a spectacular flash known as a kilonova. This violent event also leaves behind splashes of neutrons, along with heavy elements like platinum and uranium. Two neutron stars colliding can also make a kilonova, although that would stir up gravitational waves – fluctuations in the fabric of space-time itself – so, finding a kilonova without gravitational waves to accompany it could be proof of primordial black holes at work.
The black holes they detected are more massive than you’d expect from a star’s demise
There are a lot of possibilities, and some, like the neutron star scenario, are outlandish. But two methods can help us cut through the uncertainty and find more concrete proof of primordial black holes. One solid method for tracking a primordial black hole is microlensing, which happens when light from a bright object travels past something with a lot of mass. The massive object bends spacetime with its gravity and, since light follows the path of least resistance, the light from the bright object is bent, too. Even if the massive object emits no light, like a black hole, you can still measure its effect on things that do emit light. Three experiments, known as MACHO, EROS and OGLE, have done surveys of the sky looking for this celestial lensing, some of which could be from primordial black holes. These observations have made scientists pretty confident that certain sizes of PBH don’t exist.
The other method for detecting primordial black holes uses gravitational waves generated when massive objects (like black holes) disrupt spacetime. Less than a year after the groundbreaking Laser Interferometer Gravitational-Wave Observatory (LIGO) switched on its detectors in 2015, physicists detected gravitational waves from two black holes – each around 30 times larger than our Sun – spiralling in and merging with each other. Since then, they’ve found many more of these black hole mergers, opening up a plethora of new questions.
The black holes they detected are more massive than you’d expect from a star’s demise, which came as a bit of a surprise to scientists. It’s still a majority view that these larger black holes come from multiple run-of-the-mill star-sized black holes clumping together over time. But, according to Carr, several groups of scientists have argued that these big black holes could instead be primordial black holes, which could naturally form at that size.
Although scientists can’t agree on whether nearby black hole mergers are from stars or primordial black holes, finding evidence of merging black holes sufficiently far away would tip the scales towards primordial black holes. Since light can travel only so fast, when we look far away in the Universe, we’re also looking back in time. A star four light-years away is actually being seen four years in the past, since that’s how long the light we see took to reach us. Gravitational waves also can’t travel faster than light – so, if we see a black hole merger so far away that it’s from before stars formed, that would have to be a merger of primordial black holes.
There is one more observation we could make with gravitational waves that would be surefire evidence of primordial black holes: finding a black hole the size of the Sun. Stars couldn’t produce a black hole that small, so it would have to be from the early Universe.
This leads us back to our original question – if primordial black holes do exist, can they solve the problem of dark matter? Research based on Hawking radiation and microlensing rules out quite a few sizes of primordial black holes, but not all of them. There are three (or four) masses of primordial black holes that could exist and play nice with current observations, and maybe even explain the mystery of dark matter, depending on whom you ask.
Carr thinks that there are four masses of primordial black holes that could exist: asteroid-sized, moon-sized (about ~1/10th the mass of Earth), Sun-sized, and mind-bogglingly huge (more than a billion times the mass of our Sun). Although the huge primordial black holes are interesting, and may explain what we see with gravitational waves, they’re not really a candidate for dark matter. There’s also quite a bit of controversy over whether the Sun-sized ones are possible at all. That leaves asteroid- to moon-sized PBHs as the only well-accepted option to explain dark matter.
Even then, quite a few scientists are sceptical about primordial black holes, which are sometimes dismissed as a bonkers fringe idea. That doesn’t bother Kusenko at all. ‘Healthy scepticism is a welcome and necessary part of scientific discourse,’ he says. ‘So, if these folks’ scepticism is based on some logical arguments, I am happy to hear them.’
However, even he agrees that there are some cons to primordial black holes as dark matter. These small black holes are quite difficult to detect, and it’s looking somewhat unlikely that primordial black holes could make up all of dark matter given the current constraints.
More galaxies could be a sign that primordial black holes were around, helping them get started
What if they don’t have to be all of dark matter, though? A new idea gaining traction is that dark matter may be a collection of different things – an entire ‘dark sector’ that we’re just beginning to investigate. ‘If there is only One True Dark Matter, then any candidate which can’t make up the full dark matter fraction of the Universe is just not satisfactory (hello, PBH!) and can be totally ruled out,’ says Luna Zagorac, a physics PhD candidate at Yale University in Connecticut. ‘But, if it can make up any part of that fraction, then it’s harder to rule out anything [WIMPs, fuzzy dark matter, other candidates, could all be part of the mix].’
So even if primordial black holes can’t explain all of dark matter, they’d certainly have interesting effects on the Universe from the start. Beyond forming dark matter, scientists think that giant primordial black holes could help explain how the biggest black holes in the centres of galaxies – like our own Sagittarius A*, recently imaged by the Event Horizon Telescope – got started.
Constantly improving technology, moreover, should help us investigate primordial black holes. The Square Kilometre Array (SKA) telescope in South Africa, for instance, should be able to make new measurements of quickly spinning dead stars known as pulsars. Pulsars are like lighthouses, emitting huge jets of matter that flash over us as they rotate, and making it look like they blink periodically. Primordial black holes that move between a pulsar and Earth can bend that light and ever so slightly change the period of the pulsar’s blink – an effect we can observe.
New observations with optical telescopes should be able to probe for smaller primordial black holes with microlensing, too. Kusenko and collaborators, such as the physicist Misao Sasaki at the Kavli Institute for the Physics and Mathematics of the Universe in Japan, are searching for small black holes with the Subaru Telescope’s Hyper Suprime-Cam on Hawai‘i. When a small primordial black hole passes in front of a star in our nearest neighbour galaxy, Andromeda, they should be able to spot how the starlight bends around the PBH. The newly launched James Webb Space Telescope should be useful for spotting signs of PBH, too – it’ll peer deeper into the Universe’s past than ever before, finally opening a window to look at the first galaxies. If there are more galaxies or bigger ones than expected, it could be a sign that primordial black holes were around, helping galaxies get started.
Arguably the most exciting prospect for discovering black holes, though, is gravitational waves. Gravitational wave detectors could find ancient black hole mergers, black holes smaller than the Sun, or even signatures of when black holes first formed in the primordial soup of the Universe’s first second of existence. When PBHs collapsed in the very beginning of the Universe, they would have shaken up space-time – contributing to a fuzzy noise of gravitational waves known as the stochastic gravitational wave background. Our current gravitational wave detectors are all on Earth – LIGO in the United States; KAGRA in Japan; and VIRGO in Europe – but there are many more next-generation instruments on the horizon, some of which will be in space. Each of the LISA, TAIJI and DECIGO missions is a set of three spacecraft that will orbit the Sun in triangles, probing different kinds of gravitational waves than we’re able to observe here on Earth. In the coming decades, these new detectors could finally spot a definitive sign of primordial black holes. If scientists detect these exciting objects, then we could finally have a solution to at least part of the mystery of dark matter.
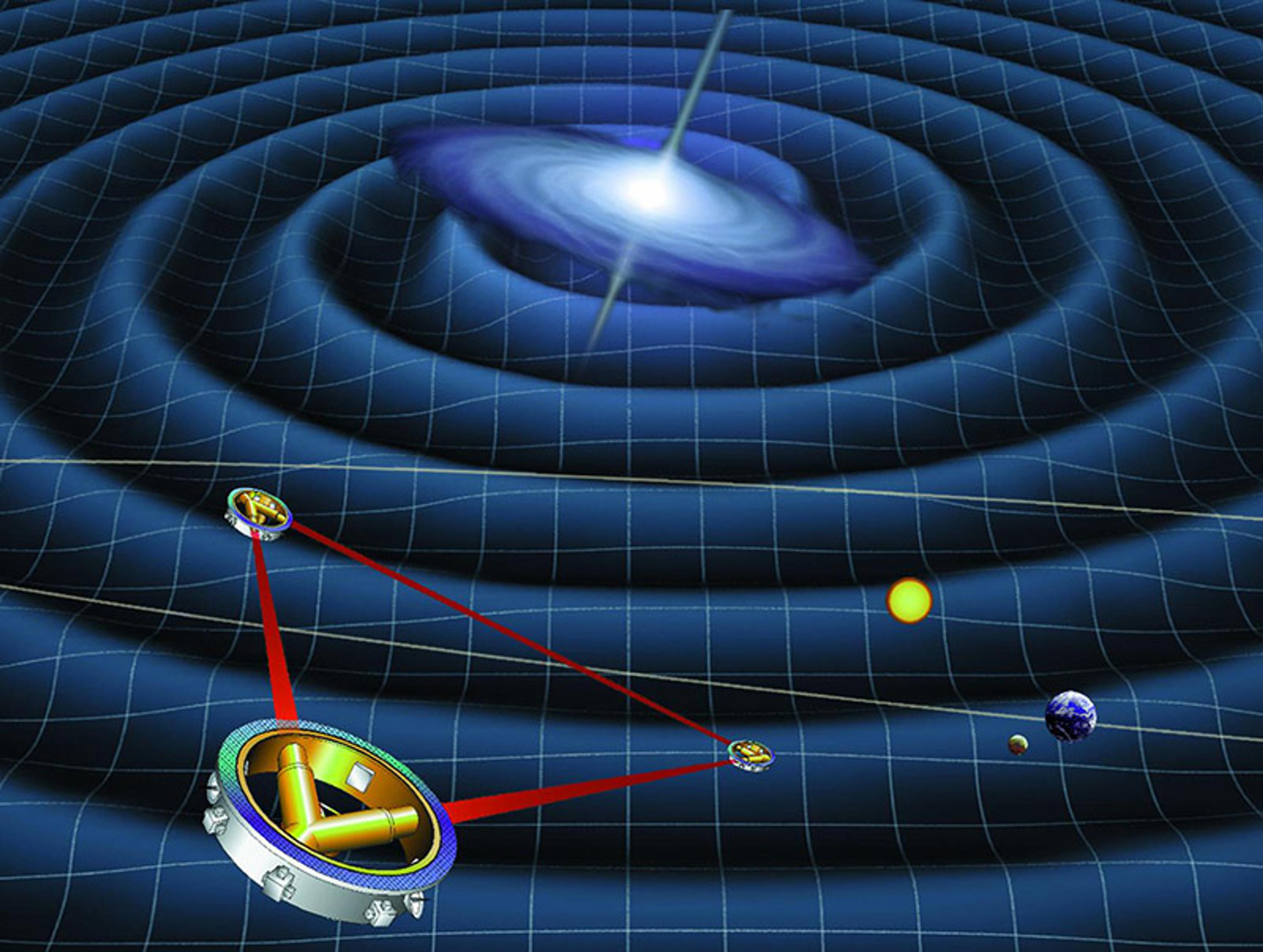
The LISA, TAIJI and DECIGO missions will orbit the Sun in triangles, probing different kinds of gravitational waves. This artist’s rendition visualises the LISA mission orbiting near Earth (not to scale). Image courtesy NASA via Wikimedia
‘PBHs are as good as the other hypothetical candidates, if not better … There are a variety of candidates for dark matter, and a lot of efforts are being made to detect them,’ says Sasaki. ‘So anyone may discover what dark matter is at any moment, maybe tomorrow.’
Carr shares this optimism: ‘I think we’re going to know fairly soon.’