As geological sites go, this one is easy to miss. It’s just a low rise of exposed rock along a back road in northern Wisconsin, outside a town whose one claim to fame is a tavern that the gangster John Dillinger used as a hideout in the 1930s. Even though I’ve been to this outcrop many times before, I drive right past it on this autumn day and need to look for a place where it’s possible for three university vans to turn around. We manage to make the manoeuvre, come back from the other direction, and park on the shoulder. Students spill out of the vehicles, clearly underwhelmed, puzzled at why we’ve bothered to stop here. They don’t yet realise that this is a secret portal into Earth’s interior.
I urge them to look closely, to get down on hands and knees for a few minutes, use their magnifying glasses, and then tell me what they see. There’s a little moaning – they’re getting hungry, and we’ve already seen a lot of rocks today – but everybody complies. Within a minute, I start to hear exclamations that make me smile: Hey, look at all that biotite! And tonnes of tiny red garnets! What are those bluish crystals – kyanite?
The students’ initial disinterest turns to respect; they understand that the rocks have taken a journey no human ever could. We’ve talked in class about rocks like these: mica schists, and their improbable biographies; how they are emissaries bearing news from alien realms. But it’s different to see them up close, in their current resting state.
By geological classification, these rocks are ‘metamorphic’, meaning that they have been transformed under punishing heat and pressure beneath the surface – and then, astonishingly, come back up. Unlike an igneous basalt crystallised from lava, or a sedimentary sandstone laid down by water, metamorphic rocks form in one environment, then go on journeys deep in the crust. This makes them the itinerant ‘travel writers’ of the rock world, returning to tell us about the restless, animate, hidden nature of the solid Earth. At each stage of their pilgrimages, they preserve a record of their experiences, and through them we can gain a glimpse of inaccessible subsurface worlds – places that we humans may never encounter directly.
The metamorphic schists my students and I are analysing in Wisconsin would have had humble beginnings as mucky, muddy sediments – the weathered residuum of still older rocks – on an ancient seabed. As more sediment blanketed and buried them, these muds lost contact with the hectic surface world, the commotion of wind and waves. Under the growing weight of the overlying deposits, the mud was compelled to let go of its natal seawater, becoming ever denser and more compact, and finally solidifying into mudstone, or shale.
Millions of years pass. The geography of the world changes with the neverending dance of tectonic plates. One day, the shale finds itself in the vice-like squeeze of colliding continents, folded deep into the interior of a mountain belt. The pressure at such depths is extreme. The fine clay minerals in the shale, now far from the shallow marine waters in which they formed, can no longer hold their shape. Their chemical bonds weaken, their grain boundaries become diffuse, and a remarkable transfiguration begins. The elements within, previously part of a rigid crystal scaffolding, are now free to wander. Atoms of aluminium, silicon, magnesium and iron, surprised at their unaccustomed mobility, form new alliances and reconfigure themselves as minerals comfortable at these depths and temperatures: shiny black biotite, wine-dark garnet, and sky-blue kyanite.
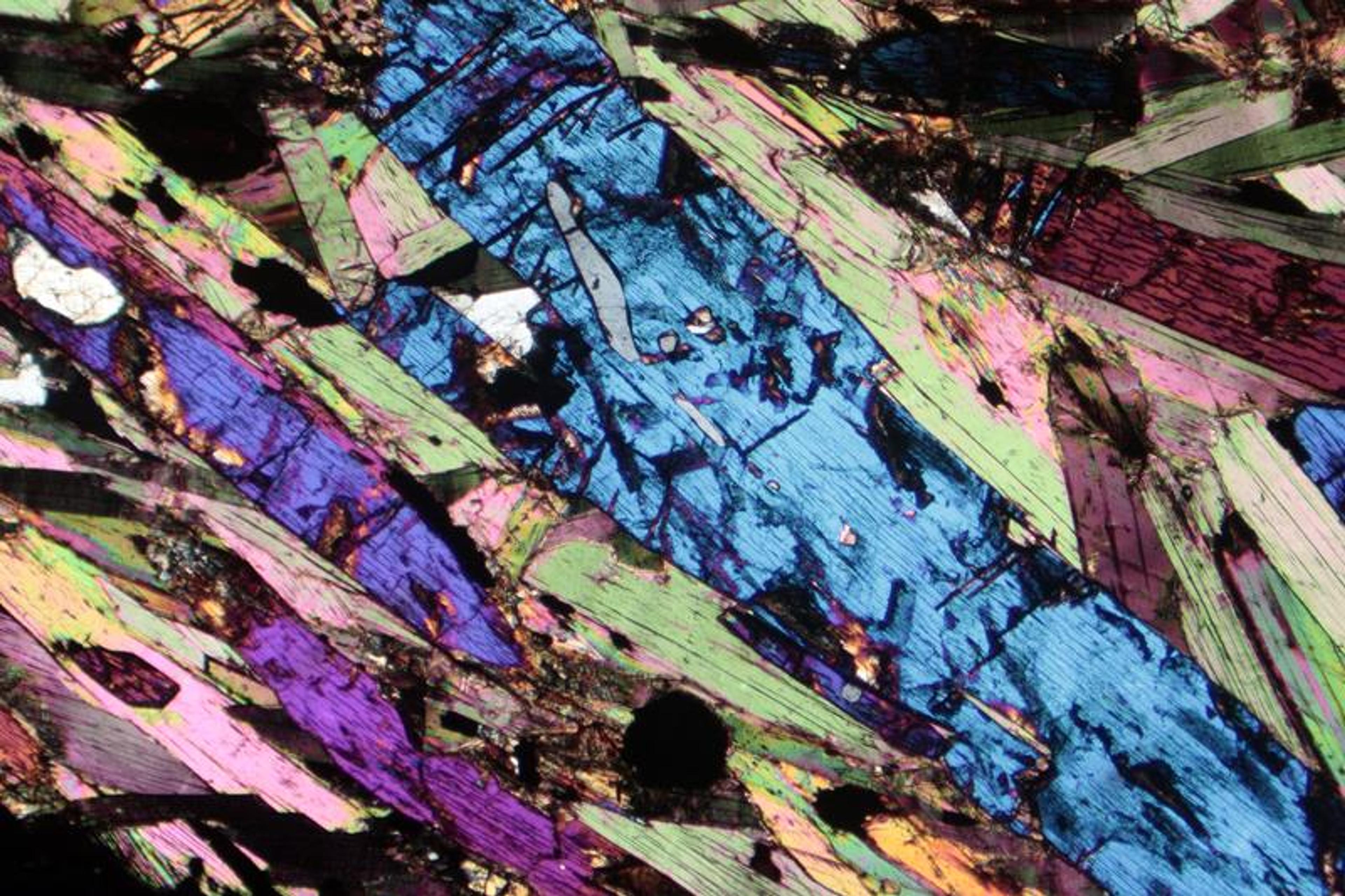
Kyanite crystals and biotite in a kyanite gneiss. Courtesy Alex Strekeisen
Intellectually, I understand the cause of metamorphism: it’s the thermodynamic imperative for crystals to reconfigure themselves into forms that are stable under new temperature and pressure conditions, in the same way that powdery snow transforms with burial to glacial ice. Still, the process strikes me as deeply mysterious, a kind of natural alchemy. Metamorphic literally means ‘after-formed’, an apt description of these shape-shifting rocks. Prosaic mud reinvents itself as resplendent mica schist, dull limestones transubstantiate into milky marbles, sandstones are reincarnated as luminous quartzites – even though in their subterranean world there is no light to reveal their beautiful new guises.
It’s like meeting the same person as a child and as an adult on one day
The mountains that harboured our schists eventually attained their maximum heights, and the tectonic action moved elsewhere. Erosion, intent on enforcing topographic egalitarianism, set to work dismantling them, focusing with special ferocity on the summits in the heart of the range. For the schists, this was the start of a slow pilgrimage back to the surface. Eventually they would feel the wind and sunlight again, testifying to anyone listening about the lofty peaks that once loomed here.
I ask my students to recall an earlier stop about 50 km to the north, where we saw some dark-grey mudstones. When I tell them those rocks were the progenitors of these garnet-biotite-kyanite schists – part of the same stratum, but never buried to such depths – they are stunned, and even strangely moved; it’s like meeting the same person as a child and as an adult on one day. Knowing what these rocks once looked like makes their journey feel more real and remarkable.
Most people would identify space as the ‘final frontier’, the last unexplored territory. But consider this asymmetry: high-altitude aircraft routinely fly 15 km above the surface of Earth, and two dozen humans have made the 384,000 km voyage to the Moon. Our satellites now clutter the outer edge of the atmosphere, and we’ve sent rovers to Mars. The Voyager 1 spacecraft left our solar system in 2012 and continues to hurtle into interstellar space. Yet no person has ever been much more than 4 km into the subsurface (the reach of the deepest mine, a gold operation in South Africa). Even our mechanical proxies haven’t gone much further. In a ridiculous Cold War competition, the USSR and NATO countries tried to best each other in deep drilling. The Soviets won that event, with a hole drilled into granites on Russia’s Kola Peninsula that managed to reach 12 km before the bit became ineffective, softened by geothermal heat. That’s little more than a long walk – about the distance from the north end of Central Park to the ferry terminals at Manhattan’s southern tip.
We do have ways of making indirect inferences about the composition of Earth’s interior – most importantly, by observing how seismic waves propagate through rocks far below the surface. But the only physical samples we have of the lower crust and mantle are metamorphic rocks, like our Wisconsin schists, that have spent time at inaccessible depths and then helpfully made the long trek back up to share with us surface-dwellers what they witnessed.
In my teaching, I sometimes struggle to convey the weirdness of metamorphism. The three main categories of rock – igneous, sedimentary and metamorphic – are akin to different literary genres. Igneous rocks are like action-packed thrillers, telling dramatic tales of volcanism and roiling magma chambers. Sedimentary rocks are serious historical tomes, admirably thorough but sometimes dull. Metamorphic rocks, in comparison, have a much greater range of narrative arcs and defy easy categorisation. Their early chapters could be igneous or sedimentary, but then the action veers in a completely different direction, with the mineral protagonists finding themselves in alien territory and having to find ways to adjust. It’s as if, midway through a scholarly biography of Lord Nelson, the scene shifts to the seafloor and he becomes Captain Nemo.
We’ve observed metamorphism – technically ‘solid-state recrystallisation’ of rocks – since at least the mid-1800s. However, our modern understanding is usually traced to work done in the 1910s and ’20s. Back then, geologists understood deep time, but they were only beginning to grapple with the idea that an internal engine fuelled by radioactive heat animates our planet. It was decades before the theory of plate tectonics would emerge and provide the explanatory framework for how mountains form and rocks could travel to new environments.
In the Scottish Highlands, geologists were mapping a complexly deformed sequence of sedimentary rocks called the Dalradian Series. We now know that these rocks record the growth of the hemisphere-scale Caledonian-Appalachian mountain chain during the assembly of Pangaea, but at that time, when continents were thought to be rooted in place, the global context of the Highland rocks was not understood. During their mapping of the Grampian Region, geologists carefully recorded the minerals in a metamorphosed mudstone. These included biotite, garnet and kyanite, the same colourful characters my students recognised in the Wisconsin schist.
At the time, geologists thought that metamorphism was caused solely by heat. So it was a surprise when they found no kyanite in similarly heated Dalradian mudstones just to the northeast of Scotland, in the Buchan district.
We can now simulate the high pressure and temperature conditions of metamorphism in a lab
Instead, the Buchan rocks were dotted with a cream-coloured mineral called andalusite, which sometimes occurs in the shape of a stubby cross. Why the difference? This seemed akin to putting two cakes made with the same batter in the oven at the same time and emerging with completely different flavours.
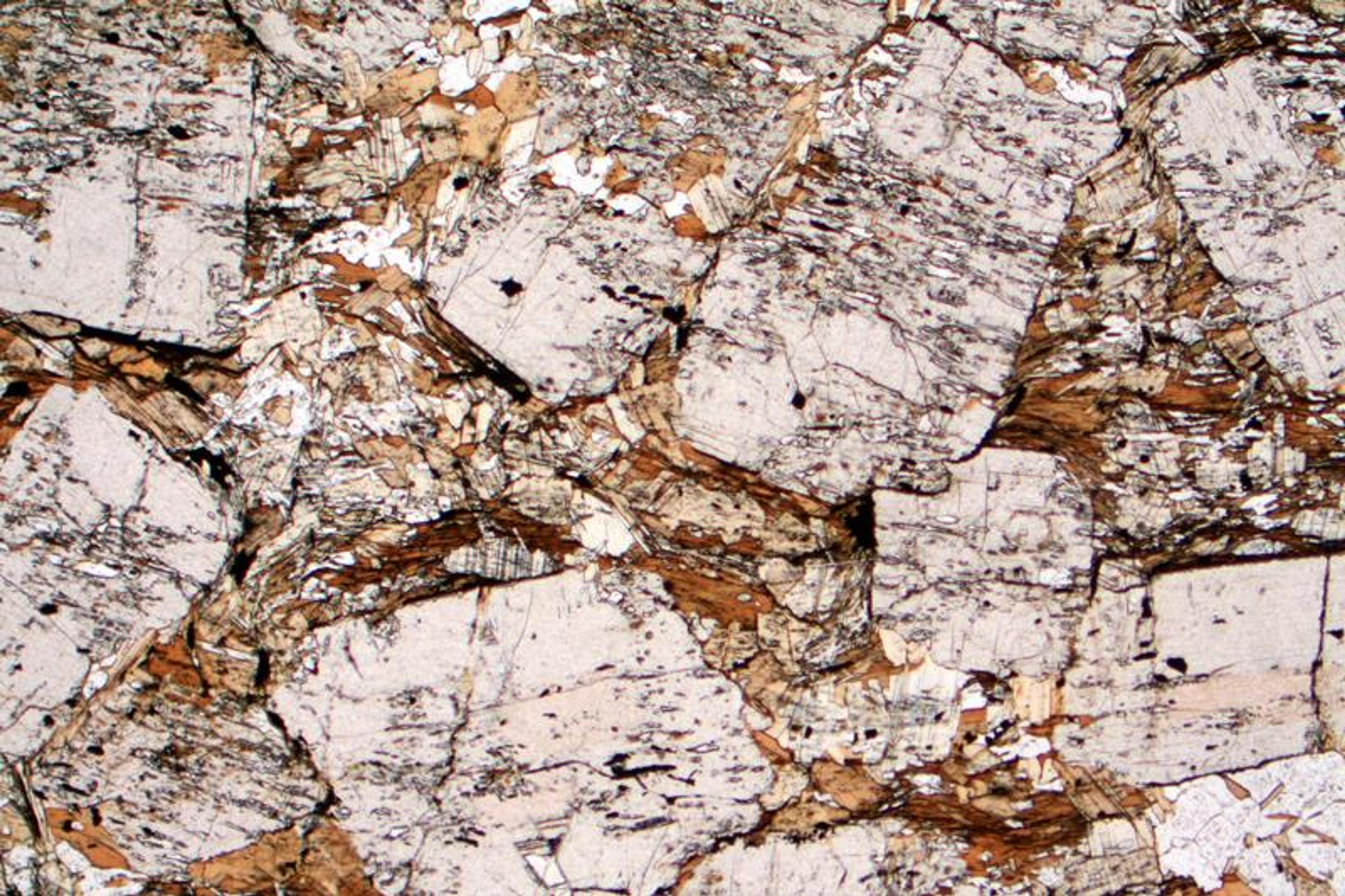
Andalusite. Courtesy Alex Strekeisen
One clue was that andalusite and kyanite have the same chemical composition and formula – Al2SiO5 – but different crystal structures. They are ‘polymorphs’ of each other, in the same way that graphite and diamond are both crystalline varieties of carbon but with distinct molecular forms. A second important observation was that kyanite is significantly denser than andalusite, indicating that its crystal structure is more compact – and formed under greater pressure. This was the solution to the Dalradian mystery: metamorphic reactions, and the metamorphic minerals that record them, are functions of both temperature and pressure.
Around the same time in the 1910s-20s, working independently, the Finnish geologist Pentti Eskola had come to the same conclusion as his British counterparts. Based on his deep knowledge of the Baltic Shield’s geology, Eskola plotted the relative positions of various metamorphic rocks on a graph of pressure vs temperature, creating the first, rough metamorphic phase diagram. He anticipated a time when the stability fields – the native habitats or ‘comfort zones’ – of metamorphic minerals could be determined quantitatively, and the obscure dialects of metamorphic rocks could be translated.
A century later, Eskola’s vision has been achieved. It’s now possible to simulate the high pressure and temperature conditions of metamorphism in laboratory experiments and coax tiny amounts of minerals like garnet, kyanite and andalusite to form. We now have detailed metamorphic phase diagrams for rocks of all starting compositions, and their travel itineraries can be reconstructed in detail.
Some metamorphic reactions – like the conversion of graphite to diamond – are primarily sensitive to pressure, with temperature playing only a secondary role. These reactions are known as ‘geobarometers’ – because the presence of one mineral or the other tightly constrains the pressures that the rocks endured. Conversely, temperature-sensitive but pressure-independent reactions are ‘geothermometers’, reliable indicators of past temperatures. Like explorers carefully keeping a log of their latitude and longitude, metamorphic rocks thus record their ‘coordinates’ in pressure-temperature space. This information, in combination with methods for dating minerals using natural radioactivity, makes it possible to track metamorphic rocks in space and time. Eskola would have been pleased and astonished.
Under the microscope, metamorphic rocks are like exquisite illuminated manuscripts, with interlacing minerals chronicling their hidden, subterranean experiences. Crystals that grew in distinct episodes over time will develop concentric bands like tree rings, and early formed minerals may be engulfed or rimmed by later ones. On the way to becoming schist, a mudstone might first have been a slate, and garnets that overgrew the slate’s aligned minerals can preserve a record of that stage even when the slaty texture has been erased in the rest of the rock – like a tree that grew around the wires of an old fence that has long since been removed.
Microscopic observations, together with laboratory experiments, have also shown that fluids in the crust – mainly water, but also carbon dioxide, and various elements dissolved in these phases – are as important as temperature and pressure in governing metamorphic reactions. Water’s presence dictates the temperatures and rates at which reactions occur, and on a watery planet this is the rule rather than the exception. Indeed, the one important scientific result of the absurd and expensive Cold War race to drill the deepest hole was the discovery that water (in a supercritical form, neither liquid nor gas) occurred even at the greatest depths reached.
Some of my own work in western Norway, another part of the great Caledonian-Appalachian mountain chain, has shown that, in the complete absence of fluids, metamorphism may not happen at all. Just north of Bergen, rocks that were once deep in the heart of that great mountain range are exposed on the ice-scoured and windswept island of Holsnøy. Tiny Holsnøy is disproportionately famous among geologists because it provides a kind of ‘counterfactual’ glimpse of an Earth in which crustal rocks are devoid of water.
It provides a ‘real-time’ view of metamorphic processes too deep in the crust for us ever to witness
The rocks on Holsnøy have the composition of basalt, the rock that makes up the ocean crust. They experienced two distinct mountain-building events, one about 900 million years ago and another around 400 million years ago – and in both cases were subjected to extreme metamorphic conditions. During the first event, the rocks experienced moderate pressures, at 25 km depth, but very high temperatures – close to their melting point – so hot that all water in hydrous minerals was ‘baked’ out, leaving an exceptionally dry rock mass.
Five hundred million years later, these parched rocks found themselves even deeper in the crust – more than 45 km down – but at less extreme temperatures. At such depth, they should have completely converted, via metamorphic recrystallisation, to a dense, improbably beautiful rock called eclogite, with raspberry garnets set in a field of grass-green pyroxene (and a particular favourite of Eskola’s). But, oddly, eclogite on Holsnøy occurs only along narrow zones and bands constituting perhaps 30 per cent of the rock mass. The eclogite-forming metamorphic reactions were for some reason frozen in action, leaving converted and unconverted rock in juxtaposition and providing a rare, close-up, ‘real-time’ view of the metamorphic processes that happen too deep in the crust for us ever to witness.
How could the rocks on Holsnøy have ignored the thermodynamic edict, under very literal pressure, to recrystallise entirely to eclogite? The answer seems to be the extraordinary dryness of the rocks at the time they were at eclogite-forming depths. Without water, atoms can move through rocks only by the arduous process of diffusion, which is tortuously slow, like driving a car in urban gridlock. Metamorphic reactions in the complete absence of water would be so sluggish that they would not happen even on geologic timescales. In contrast, when water is present, atoms can slip into solution and hitch a ride, akin to hopping on the subway and moving swiftly through the city. Transported quickly to new sites, the elements in rocks can easily reorganise themselves, and build minerals that are stable under the new conditions.
We think that, on Holsnøy, eclogite-forming reactions were suppressed by the lack of water until several large earthquakes shattered the unusually dry rocks and allowed limited amounts of external fluids to enter along fractures, forming the localised lenses of eclogite that are so odd and remarkable. Equally remarkable is the fact that these rocks ever made it all the way back to the surface, via tectonic uplift and tenacious erosion, to be marvelled at by human pilgrims.
This might seem an arcane story about weird rocks from a remote place, but it illuminates some fundamental truths about Earth’s plate tectonic system, and in particular its signature process of subduction. Along subduction zones, like the one sitting off Japan’s east coast, basaltic ocean crust plunges into the mantle. Subduction of the ocean floor is Earth’s brilliant recycling and rejuvenation system, and without it our planet would be utterly different.
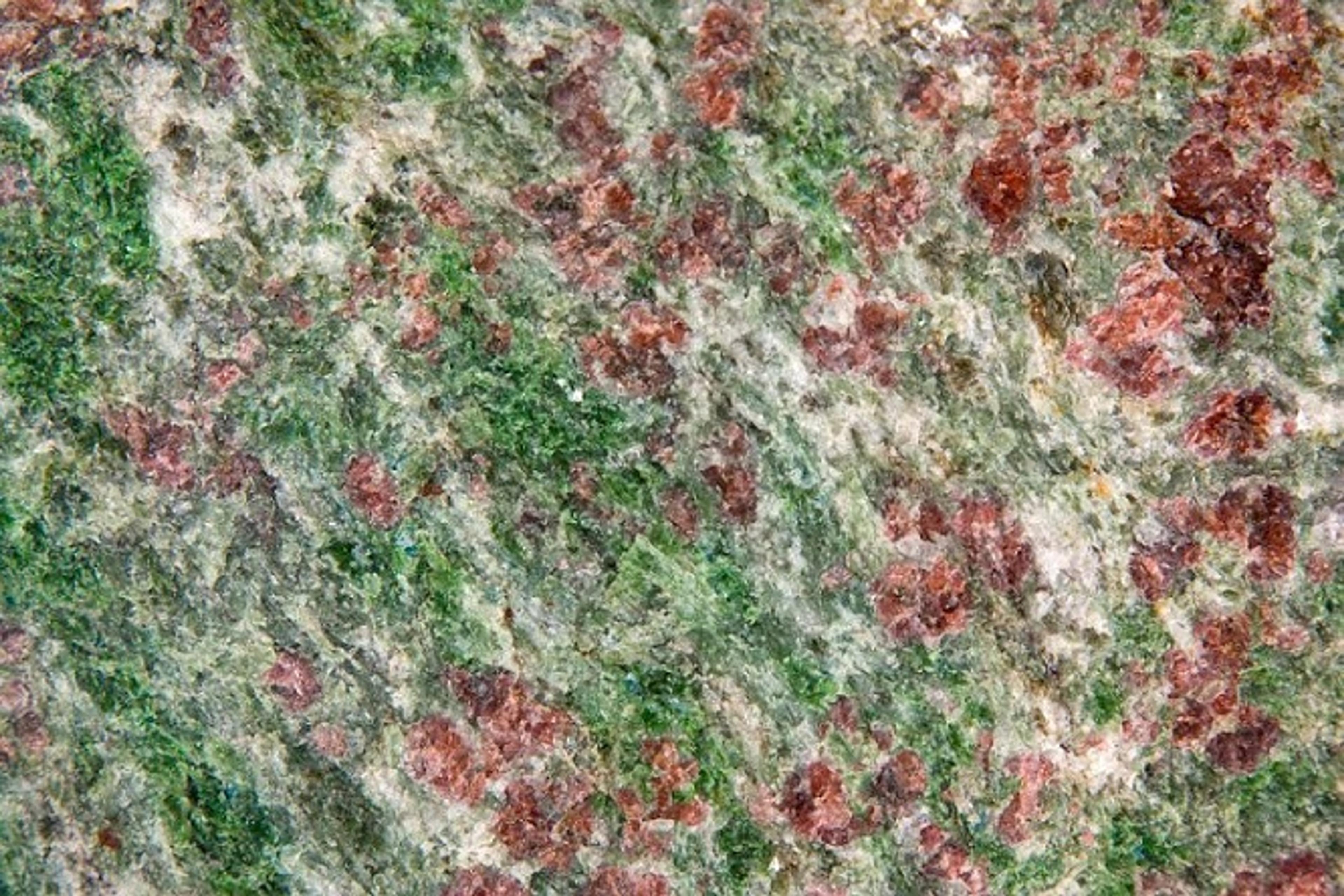
Eclogite from western Norway. Courtesy Alex Strekeisen
However, slabs of ocean crust would be too buoyant to sink very far into the mantle if they did not convert to their much denser metamorphic form: eclogite. To me, one of the strangest things about Earth is that basalt – which is derived from the mantle – can be recrystallised at high pressure into eclogite, which is denser than the mantle. Our work on Holsnøy suggests that, in the absence of water, the basalt-eclogite conversion would not happen, deep subduction would be impossible, and the planet’s tectonic system would be utterly different. Water-mediated metamorphism, in other words, is the key to plate tectonics, which rejuvenates topography, replenishes the atmosphere, and keeps the planet in a constant state of renewal.
Only Earth rocks have the chance to study abroad, wander the globe, dive into the crust and recreate themselves
More generally, given the crucial role of water in their formation, metamorphic rocks of any type (except those created by brute force in meteorite impacts) may be unique in the solar system. And in the absence of plate tectonics, which is also unique to Earth, opportunities for rocks to travel to new environments, and thereby be transformed, would be limited anyway. Moon rocks collected in the Apollo missions, and rare meteorites that have come to us from Mars, attest to our harrowing bombardment by space debris but have no stories to tell about adventures on their own worlds. Despite their long trips to Earth, these rocks are strangely naive, inexperienced.
Only Earth rocks have the chance to study abroad, wander the globe, dive into the crust and recreate themselves. In fact, metamorphic rocks are actually the dominant type on Earth, because most rocks, if they are around long enough, will find themselves in new environments.
It is our good fortune to have such an abundance of metamorphic rocks in our midst, with insights to share not only about their origins but everything they’ve experienced since. It’s not too much of a stretch to say that they are responsive, even sentient, in that they ‘perceive’ their surroundings and change in response. Their stories are genuinely epic: the journey of a rock like our Wisconsin schist from the surface to the centre of a mountain belt and back echoes the narrative arc of katabasis and anabasis in Greek myth: the protagonist’s descent into the Underworld, the tribulations experienced there, and the eventual return, with hard-won wisdom, to the land of the living.
Metamorphic rocks embody the animate, resilient, creative nature of the solid Earth. For us mortal Earthlings, they speak of the possibility of reinvention, the beauty of transformation – and the ephemerality of any particular version of the world.
It’s getting late, almost time to leave. In the slanting rays of the late afternoon sunlight, a sense of serenity fills me as I watch students crawling across this easily missed outcrop of mica schist on their hands and knees, like supplicants approaching a holy shrine.