Some months ago, I went through a bunch of (virtually) piled-up research papers from my computer’s ‘important s*%t’ folder. (Please, authors of those papers, do not feel offended. I tend to name my folders in specific ways, and I guess I am not alone in this habit.) I needed some free space, and we are talking about more than some gigabytes of data. Out of curiosity, I scanned the long list of studies I’d been saving for later reading for approximately a decade now, promising myself many times that I would undoubtedly read them when I had a little break in my research and teaching duties. Doing so, I accidentally ran into a paper from 1973 that changed the direction of my research and the way I think about our origin and existence.
The study asked the same question I asked my younger self decades ago: what if life evolved on other planets first and had been somehow directed to seed early Earth? Finding that study made me think about the topic again, but now, with additional knowledge compared with my younger self, I try to resolve at least some components of the dilemma. Was there a ‘ground zero’ planet (or planets) where life evolved and later swarmed out to the Universe – and had it arrived by natural means, or had it been sent by a civilisation more advanced and ancient than our own?
The idea goes all the way back to the Greek philosopher Anaxagoras, who used the term ‘panspermia’ in the 5th century BCE to invoke the concept of travelling between planets as seeds. More recently, the Swedish scientist Svante Arrhenius, one of the founders of physical chemistry, suggested the idea of microscopic spores’ transportation through interplanetary space in his book Worlds in the Making: The Evolution of the Universe (1906). But the modern form of the theory originates from one of the pioneers of DNA, the Nobel prize laureate Francis H C Crick, and Leslie E Orgel, a chemist famous for building theories about the origin of life. Their idea, called ‘directed panspermia’, suggests that life could have been deliberately transferred to Earth by intelligent beings from elsewhere in the Universe. First presented at a scientific conference and then published in the scientific journal Icarus in July 1973, it soon became grist for science fiction, too.
Along with Crick and Orgel, there have been many scientists influenced by the idea of panspermia. Among the most prominent, the astronomers Fred Hoyle and Chandra Wickramasinghe became central figures for the revival of panspermia around the late 20th century. Since this revival, many aspects of the theory have been investigated by the most up-to-date scientific methods available, attracting the next generation of scientists to the fold.
The same year that Crick and Orgel published their concept in Icarus, Big Ear – the radio telescope of Ohio State University Radio Observatory – first turned toward the sky for SETI, the scientific search for extraterrestrial intelligence, a programme originally funded by NASA. A few years later, on 15 August 1977 to be exact, Big Ear was eavesdropping on the ‘water hole’ – not so much a location as a band of the electromagnetic spectrum in interstellar space, where emissions from natural hydrogen (H) and hydroxyl radical (OH) gases combine to form water. According to Project Cyclops, one of the first handbooks for detecting extraterrestrial life, the water hole is a relatively quiet, noise-free channel that would be perfect for interstellar and intergalactic communication between intelligent species, should they want to engage.
At 22:16 Eastern Standard Time on the infamous date, the silence of the water hole broke for 72 long seconds. The signal detected by the radio telescope peaked at 30 times the level of the static radio noise always present.
One person in particular was thrilled. Jerry Ehman, a local physicist who volunteered at Big Ear out of interest and enthusiasm, discovered the sequence of letters and numbers output by the computer – 6EQUJ5, a code that indicated their strength and changing intensity over time. The starting number of the series, 6, shows us that something stronger than the regular noise arrived at our radio telescope. We might think that it is just some error, but by translating the language of early radio telescopes, we already know that the series of letters E, Q and U indicates that the signal got stronger and stronger. Succeeding this uniquely strong peak, the signal then fades away into the well-accustomed monotony of radio static, shown by J and 5, the closing members of the presumptive ‘code’. Ehman immediately understood its implication, and in those excited, elevated moments, he quickly scribbled a side note on the paper and unintentionally named this strong, unique and not-yet-repeated mysterious radio signal ‘Wow!’ Could the signal that broke the monotony of the water hole’s radio static have originated from intelligent beings on another world?
Suppose life was planted on Earth by an intelligent extraterrestrial civilisation
And if so, why? From novels such as Arthur C Clarke’s 2001: A Space Odyssey (1968) to Carl Sagan’s Contact (1985), the question of why such beings would reach out over the vastness of the cosmos have arisen again and again. It could be that such a signal is a torchlight, a signpost telling the ones who accidentally received it: ‘We are here, ready to make contact,’ similar to the two identical copies of the Golden Record, a greeting from Earth carried by the Voyager spacecraft into the void.
In short, the insight of Ehman and the Big Ear scientific team resonated with the hypothesis of directed panspermia, introduced by Crick and Orgel just four years before. Suppose life was planted on Earth by an intelligent extraterrestrial civilisation. In that case, such a society must be capable of targeting, and sending life to, our planet, for example, in the form of complex organic molecules or microbes implanted in some object that may survive travel through the galaxy. A civilisation sophisticated enough to send a radio message to Earth would be an excellent candidate to shoot an asteroid filled with the bricks of life toward the early Earth.
I bet I am not alone in thinking that finding even just a microscopic sign of life in any relic from space would be a giant leap for humankind. That is most likely what scientists in the mid-1990s felt when examining a Martian meteorite named Allan Hills (ALH) 84001. A nanometres-sized chain of magnetic minerals appeared on the screen connected to a scanning electron microscope, a special instrument capable of showing objects the size of dust particles floating around us. Such minerals looked very similar to the so-called magnetosome, magnetic iron-bearing nanoparticles that function as tiny compasses in the cells of a particular type of bacteria.
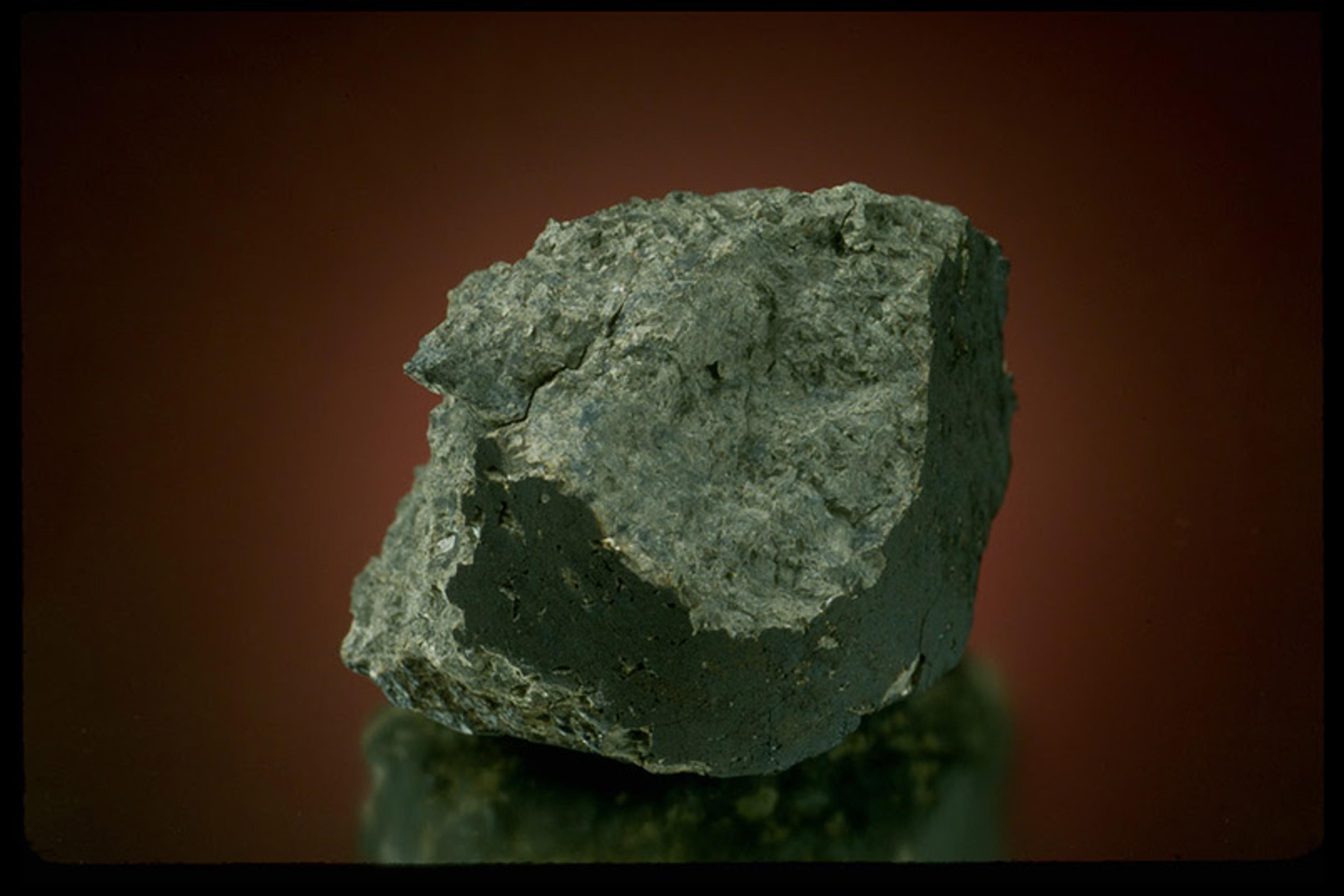
The meteorite Allan Hills (ALH) 84001. Courtesy Smithsonian National Museum of Natural History
From the moment they saw those mineral chains, the group of scientists involved in the ALH enigma divided into two. Some believed in the biological nature of the mineral chain and defined it as an extraterrestrial magnetofossil from the past of Mars. Others denied the biological origin of the relic and explained its formation in alternative ways, such as abiotic organic synthesis and artificial laboratory artefacts. Even today, disputes repeatedly flare up around the organic or inorganic origin of such microscopic crystal chains.
Despite all the back and forth about evidence for microscopic forms of life on other worlds, panspermia theory itself was pushed back from the centre of interest for a decade – until the Cassini spacecraft arrived at Saturn in 2005 and sent the first close-up images of Enceladus, one of the gas giant’s icy moons, back to Earth. Enceladus revealed his secret, which was kept hidden for a billion years: flying close over its surface, Cassini captured cracks – nicknamed ‘Tiger Stripes’, because they resemble the animal’s marks – around the moon’s south pole and, more importantly, its geysers spraying out from the fissures, illuminated by the dim light of the distant Sun. Water plumes of ice and vapour mixed with essential compounds of more complex organic materials. Such water jets, studied by the Cassini spacecraft, may be linked to underground water reservoirs originating from an ocean hidden under Enceladus’s frozen surface, containing key chemical elements of many biological processes abundant in the subsurface ocean.
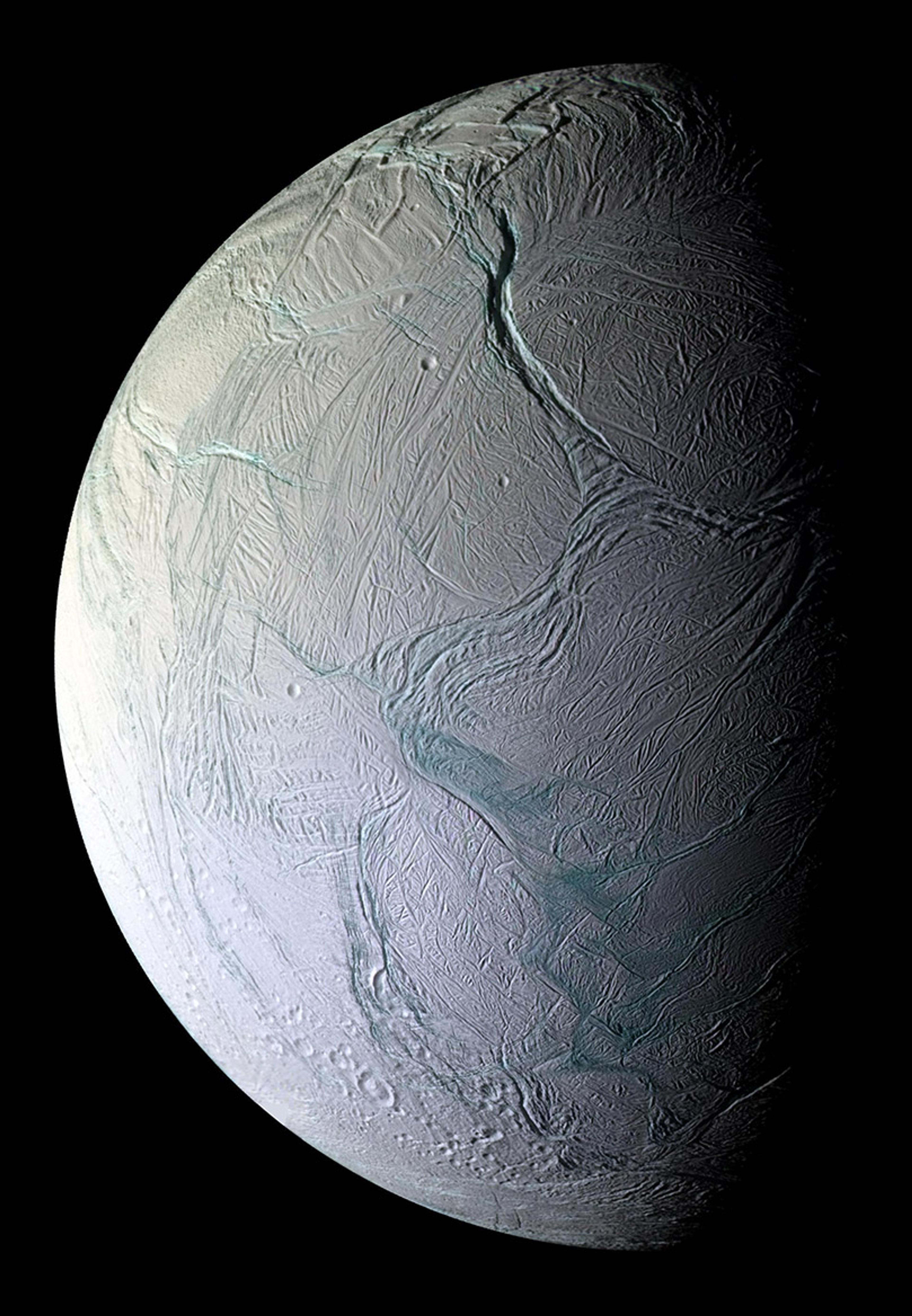
Cassini took this photograph of Saturn’s moon Enceladus from within 25 km of the moon’s surface on 9 October 2008. NASA/JPL/Space Science Institute
With the finding, panspermia struck back, and the theory again grabbed our attention. The news broke and spread like a streak of lightning, not limited to the scientific community: icy worlds could allow biological evolution and harbour life in their hidden oceans below the surface. I wondered whether such worlds were actually incubators for primordial life, poised to spread across the cosmos. Were icy moons points of origin for life hopscotching from world to world, stored for posterity under the surface but ready to travel aboard a meteor or some other celestial object to a place like Earth? If so, was this clever system an accident of the Universe, or by design? Were such moons and other icy bodies the ultimate source of life? Why not? Panspermia is believed to be universal. Whether life was spread intentionally or not, the real question is where and when the first living entities had a chance to evolve. Did life actually evolve anew on Earth, or are icy worlds, distant and nearer, really ‘ground zero’ for the origin of life?
What if microbial life swarms out from under the icy shell of ancient moons?
Based on our commonly accepted, Earth-based, geocentric knowledge, water is one of the essential requirements for the first steps of biological evolution. Down at the ocean bottom, fissures open and warm up the water with the heat of the magma lying deep below. Along those vents, active hydrothermal processes in the re-emerging hot water provide a key, mineral-, compound- and element-rich environment to prebiotic synthesis, when the building blocks of first cells are formed.
Water might have already been abundant in the epoch cosmologists call ‘first metal enrichment’, when, astronomically speaking, many elements heavier than hydrogen and helium formed by nuclear fusions in the earliest existing stars. Ranging between 10 and 1,000 times our Sun, these extremely massive, ginormous hydrogen and helium-built stars, referred to as Population III (or Pop III) by astronomers, took seriously the injunction to ‘live fast, die young’ and ejected their material into space at the last moments of their short, few-hundred-million-years life cycle. While they exhausted their primordial, hydrogen- and helium-containing gas fuel, they produced elements heavier than the two gases, which elements are referred to as ‘metal’ by astronomers. However, many of those ‘metals’, such as carbon and oxygen, are not considered as metals in common sense. Theoretically, Pop III stars’ short but very productive life enriched the cosmos with elements, creating the abundance of water in the interstellar medium at the time when the Universe was only approximately 300-400 million years old. At least one study says that the habitable cosmological epoch, in which life could evolve on ocean-bearing rocky planets, emerged when our 13.8 billion-years-old Universe was only 10-17 million years old.
Real-life observations provide additional support for such theoretical calculations. One of the oldest observed planets, PSR B1620-26 b (sounds like a droid from Star Wars), often called Planet Methuselah, is approximately 12.7 billion years old. Another great old one, WASP-183 b, is thought to be at least 13 billion years old.
Both ancient worlds are gas giants like our far planetary neighbour, Jupiter, known for its great red spot and its four Galilean moons: Io, the home of volcanoes, and three with an icy shell and a putative subterranean ocean perhaps harbouring life: Europa, Ganymede and Callisto.
What if the earliest planets in the Universe, those Jupiter-like gas giants, have their own icy moons with subterranean oceans that could also evolve and harbour life? What if microbial life swarms out from under the icy shell of those ancient moons, catches a ride on a meteor, and fertilises our entire Universe?
The ‘small’ WASP-183 b, half the size of Jupiter, is orbiting around a G-type star, a host like our Sun. Unfortunately, the similarities end there. The distance of the ancient planet from its Sun-like host star is a fraction of the distance between the Sun and Mercury. Even if we wish for a Europa- or Ganymede-like icy moon around the gas giant as a potential life-harbouring place, water – a possible key compound of ocean formation and life – cannot stay in the liquid or solid phase in the scorching heat at such close distance to a host star.
This led me to Methuselah, more than twice as big as Jupiter and living in a binary star system of pulsar and white dwarf. Despite the size difference and the binary suns, similarities could abound – especially if we look back in cosmological time.
Originally, Methuselah was born out of a protoplanetary disk, swirling around a Sun-like progenitor star in a similar setting to the Sun and Jupiter. Later, the star system was captured by a neutron star and its companion. During such an exchange, the couple broke up, and the companion of the neutron star was left behind. At the start of a new chapter in the life of the neutron star, a fresh relationship was formed when the host of Methuselah became the latest member of the binary system. Time passed, and the Sun-like star turned into a red giant, feeding the neutron star (later, a pulsar) with its material, and ending up as a white dwarf. Yet Methuselah witnessed this transformation at a safe distance and continued its orbit undisturbed around the binary star system that served as its new host. A theoretical icy satellite of Methuselah, similar to the moons of Jupiter, is still a candidate for the origin of life. Could such icy satellites be the ground-zero locations of life in the Universe?
Stored at depth, life would be protected from electron bombardment in the radiation belts around Jupiter
There needs to be more than just a subsurface ocean to keep the potential lifeforms alive, of course. The environment must be oxygenated, like the oceans on Earth. And geological renewal processes like tectonism and volcanism, which promote communication between the subsurface ocean and the icy surface, would be needed to convey the oxidants and other materials needed for life below.
To see how this works, look no further than the surface of Europa with its labyrinthine pattern of linear features: long, curvy lineaments run through half of the moon, accompanied by thin lines, barely visible even on the highest-resolution spacecraft images. There are troughs and ridges evolving into more complex patterns, including double ridges and ridged bands. The chaotic pattern of those lineaments shows how the surface repeatedly renews by exchanging material with the ocean below.
The presence of the subsurface ocean and the possibility of extraterrestrial life has made the Jovian moons the primary targets of ongoing outer solar system explorations. The Jupiter Icy Moons Explorer (JUICE) is already on its eight-year journey to the Jovian moons.
Europa Clipper is in the last moments of its preparation phase, with a planned launch in autumn 2024. During close flybys over Europa’s frozen surface, the spacecraft has one overall objective: to determine if Europa can support life in the subterranean ocean below its icy shell.
Succeeding those missions to collect data by flying around the icy satellites, a new challenge awaits. Europa Lander, still in the concept stage, would land on Europa’s surface to directly sample it and search for potential traces of life, so-called biosignatures, 10 centimetres below the icy surface. Stored at such depth, traces of life with complex chemistry would be protected from the damaging high-energy electron bombardment in the radiation belts around Jupiter.
A swarm of wedge-shaped mini-robots will swim through the subsurface ocean and explore
Once we can land on the surface of an icy satellite, we could design an instrument capable of penetrating the crust and reaching the ocean tens of kilometres beneath the crust. On Earth we execute such exploratory ventures by building drilling rigs to an enormous depth, then adjusting the drill pipe’s length to the hole’s growing depth. Unfortunately, the mass and volume of these instruments would be prohibitive aboard spacecraft bound for icy moons. In addition, you’d still need a human to operate those instruments. Imagine the challenge of transporting elements and building a 10-storey construction capable of drilling through the icy crust of Europa. We definitely and desperately need an alternative to succeed, and that is where cryobots, which can travel aboard spacecraft, come in.
The concept of cryobots to penetrate the ice shell and investigate Europa’s hidden ocean has been developed through NASA’s Scientific Exploration Subsurface Access Mechanism for Europa (SESAME), which aims to open the treasure chest of life hidden under the satellite’s icy surface.
The cryobots under consideration are robot probes with an array of telling names: SLUSH (from Honeybee Robotics), VERNE (from Georgia Tech), and the late VALKYRIE, now SPINDLE (from Stone Aerospace). These small explorers, working together, will melt the icy crust and dig through the surface using a variety of thermal sources along with drilling and cutting instruments and waterjet assembly. Make way!
Following on the heels of the cryobots, a swarm of wedge-shaped mini-robots called SWIM (Sensing With Independent Micro-swimmers), a dozen centimetres in length, will patiently wait to swim through the subsurface ocean and explore. Meanwhile, BRUIE (Buoyant Rover for Under-Ice Exploration), another type of device, will rise to the undersurface of the ice crust, wandering ‘upside down’ to search for life at the boundary of water and ice. When these systems are finally deployed, they should be able to provide highly anticipated direct evidence of extraterrestrial life, at last.
Now, it’s time to connect the dots. The panspermia theory suggests that life on Earth might originate from an extraterrestrial source. At least for me, panspermia is strongly connected to our neverending search for the origin of humankind. We want to know where we came from. We want to feel how deeply our roots penetrate across space and in time.
Evolutionary scientists search for human roots in geologic time, often looking to the Miocene, a period marked by the disappearing remains of an ancient ocean called Tethys. It was an epoch where the peaks of the transcontinental Alpine-Himalayan mountain chain rose and, toward the end, in a continuously cooling global climate, where the Antarctic ice sheet loomed larger. If we look closer, we may find a creature following a hidden path in the trees of a tropical-subtropical deciduous forest, heading toward a more open area with the hope of fruit- and insect-containing lunch on the way. Some say it looked like an ancient ape, and others thought it was more like a modern gorilla or chimpanzee. Still, both agree that its appearance around 8 to 6 million years ago marks a critical moment in biological evolution: the dawn of humankind. The creature is called the Last Common Ancestor or Homo-Pan, indicating the spot on the evolutionary tree of life where the branches of the hominin and the pan (ancestors of the chimpanzee and the bonobo) lineages diverged.
Going back in time, all converging branches of the phylogenetic tree led to one common ancestor of all cells – referred to as Last Universal Common Ancestor (LUCA). Even though some scientific studies separate the first living cell and LUCA, the latter is often referred to as the evolutionary intermediate connecting early Earth’s abiotic world with the period when microbial life, preserved in rocks as fossils, appeared on the surface of Earth around 3.5 billion years ago. In the quest to find our most ancient roots, this milestone is the one we can say out loud, without being labelled a heretic or a joke.
Microbes could hitch a ride on an asteroid and travel through the vast nothingness of space
But did LUCA emerge from the molecules formed by more and more complex networks of chemical reactions in the abiotic world here on Earth – or did LUCA arrive from space?
It’s possible that molecules and compounds resulting from chemical evolution, the ones responsible for creating the building blocks of life, may appear not only on Earth but on Mars and the icy moons.
According to recent experiments aboard the International Space Station, such molecules can survive the vicissitudes of space. Microbes, along with complex organic molecules (the precursors of the first living cells), would be able to hitch a ride on an asteroid and travel through the vast nothingness of space to arrive somewhere else intact. With any luck, those newcomers would survive more than a bumpy landing on a new planet; arriving at their new home, they would embark upon biological evolution anew.
What if some of those asteroids are artificial, made by an intelligent civilisation and sent through interstellar space to fertilise other worlds? From UFO buffs to SETI enthusiasts to some scientists, the notion of directed panspermia is still very much in play. One potential source could be the oblong-shaped asteroid ‘Oumuamua (Hawaiian for ‘scout’), an interstellar visitor that the Harvard physicist Avi Loeb contends might have been propelled to our solar system like a light sail. (Most of his colleagues disagree.) Loeb and his team do not stop at ‘Oumuamua. Their search for an interstellar visitor also points to CNEOS 20140108, an interstellar meteor that fell into the South Pacific and is now referred to by some folks as Interstellar Meteor 1. As recently as this summer, Loeb’s team used a powerful magnet to dredge the bottom of the ocean around what they think is the impact location, analysing some 50 microscopic iron spherules they suspect could be the meteor’s remains. If the spherules turn out to be artificial (of course, a big if), it would support the directed panspermia theory and revolutionise our knowledge of the Universe.
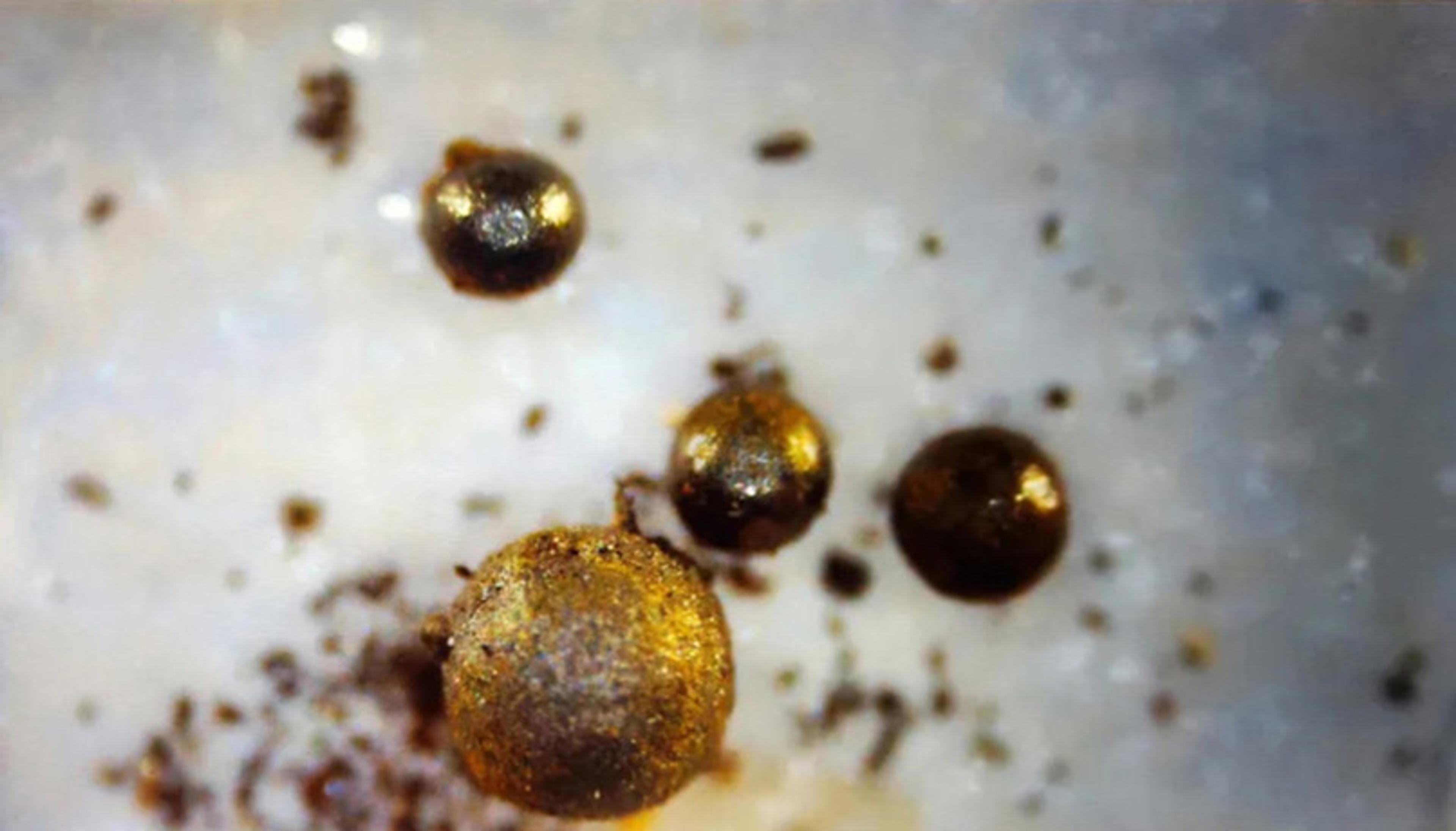
Tiny meteoritic spherules from the most likely path of Interstellar Meteor 1. Courtesy Avi Loeb, Harvard University/Galileo Project
But you don’t need an alien to send life through space, perhaps from a theoretical icy moon of Methuselah. If we can prove the incubation of life on the icy moons of Jupiter, that would offer a natural means of seeding LUCA through space. Given evidence that such forms could survive this kind of deep-space journey, the idea of a Last Intergalactic Universal Common Ancestor may not be far fetched.
This Essay was made possible through the support of a grant to Aeon+Psyche from the John Templeton Foundation. The opinions expressed in this publication are those of the author and do not necessarily reflect the views of the Foundation. Funders to Aeon+Psyche are not involved in editorial decision-making.